DNA structure-induced recruitment and activation of the Fanconi anemia pathway protein FANCD2
- PMID: 17420278
- PMCID: PMC1900049
- DOI: 10.1128/MCB.02196-06
DNA structure-induced recruitment and activation of the Fanconi anemia pathway protein FANCD2
Abstract
The Fanconi anemia (FA) pathway proteins are thought to be involved in the repair of irregular DNA structures including those encountered by the moving replication fork. However, the nature of the DNA structures that recruit and activate the FA proteins is not known. Because FA proteins function within an extended network of proteins, some of which are still unknown, we recently established cell-free assays in Xenopus laevis egg extracts to deconstruct the FA pathway in a fully replication-competent context. Here we show that the central FA pathway protein, xFANCD2, is monoubiquitinated (xFANCD2-L) rapidly in the presence of linear and branched double-stranded DNA (dsDNA) structures but not single-stranded or Y-shaped DNA. xFANCD2-L associates with dsDNA structures in an FA core complex-dependent manner but independently of xATRIP, the regulatory subunit of xATR. Formation of xFANCD2-L is also triggered in response to circular dsDNA, suggesting that dsDNA ends are not required to trigger monoubiquitination of FANCD2. The induction of xFANCD2-L in response to circular dsDNA is replication and checkpoint independent. Our results provide new evidence that the FA pathway discriminates among DNA structures and demonstrate that triggering the FA pathway can be uncoupled from DNA replication and ATRIP-dependent activation.
Figures
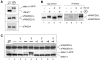
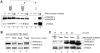
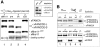
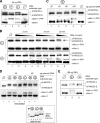
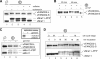
Similar articles
-
The Fanconi anemia protein FANCM is controlled by FANCD2 and the ATR/ATM pathways.J Biol Chem. 2009 Sep 18;284(38):25560-8. doi: 10.1074/jbc.M109.007690. Epub 2009 Jul 24. J Biol Chem. 2009. PMID: 19633289 Free PMC article.
-
Fanconi anemia proteins are required to prevent accumulation of replication-associated DNA double-strand breaks.Mol Cell Biol. 2006 Jan;26(2):425-37. doi: 10.1128/MCB.26.2.425-437.2006. Mol Cell Biol. 2006. PMID: 16382135 Free PMC article.
-
Fanconi anemia proteins FANCD2 and FANCI exhibit different DNA damage responses during S-phase.Nucleic Acids Res. 2012 Sep 1;40(17):8425-39. doi: 10.1093/nar/gks638. Epub 2012 Jun 29. Nucleic Acids Res. 2012. PMID: 22753026 Free PMC article.
-
A novel cell-free screen identifies a potent inhibitor of the Fanconi anemia pathway.Int J Cancer. 2009 Feb 15;124(4):783-92. doi: 10.1002/ijc.24039. Int J Cancer. 2009. PMID: 19048618
-
The interplay of Fanconi anemia proteins in the DNA damage response.DNA Repair (Amst). 2004 Aug-Sep;3(8-9):1063-9. doi: 10.1016/j.dnarep.2004.04.005. DNA Repair (Amst). 2004. PMID: 15279794 Review.
Cited by
-
Monoketone analogs of curcumin, a new class of Fanconi anemia pathway inhibitors.Mol Cancer. 2009 Dec 31;8:133. doi: 10.1186/1476-4598-8-133. Mol Cancer. 2009. PMID: 20043851 Free PMC article.
-
The genetic and biochemical basis of FANCD2 monoubiquitination.Mol Cell. 2014 Jun 5;54(5):858-69. doi: 10.1016/j.molcel.2014.05.001. Mol Cell. 2014. PMID: 24905007 Free PMC article.
-
Fanconi anemia proteins stabilize replication forks.DNA Repair (Amst). 2008 Dec 1;7(12):1973-81. doi: 10.1016/j.dnarep.2008.08.005. Epub 2008 Sep 25. DNA Repair (Amst). 2008. PMID: 18786657 Free PMC article.
-
Regulation of FANCD2 and FANCI monoubiquitination by their interaction and by DNA.Nucleic Acids Res. 2014 May;42(9):5657-70. doi: 10.1093/nar/gku198. Epub 2014 Mar 12. Nucleic Acids Res. 2014. PMID: 24623813 Free PMC article.
-
The Fanconi anemia protein FANCM is controlled by FANCD2 and the ATR/ATM pathways.J Biol Chem. 2009 Sep 18;284(38):25560-8. doi: 10.1074/jbc.M109.007690. Epub 2009 Jul 24. J Biol Chem. 2009. PMID: 19633289 Free PMC article.
References
-
- Aquiles Sanchez, J., D. R. Wonsey, L. Harris, J. Morales, and L. J. Wangh. 1995. Efficient plasmid DNA replication in Xenopus egg extracts does not depend on prior chromatin assembly. J. Biol. Chem. 270:29676-29681. - PubMed
-
- Blow, J. J., and R. A. Laskey. 1986. Initiation of DNA replication in nuclei and purified DNA by a cell-free extract of Xenopus eggs. Cell 47:577-587. - PubMed
-
- Ciccia, A., C. Ling, R. Coulthard, Z. Yan, Y. Xue, A. R. Meetei, H. el Laghmani, H. Joenje, N. McDonald, J. P. de Winter, W. Wang, and S. C. West. 2007. Identification of FAAP24, a Fanconi anemia core complex protein that interacts with FANCM. Mol. Cell 25:331-343. - PubMed
-
- Conn, C. W., A. L. Lewellyn, and J. L. Maller. 2004. The DNA damage checkpoint in embryonic cell cycles is dependent on the DNA-to-cytoplasmic ratio. Dev. Cell 7:275-281. - PubMed
Publication types
MeSH terms
Substances
Grants and funding
LinkOut - more resources
Full Text Sources
Research Materials
Miscellaneous