DNA-dependent protein kinase inhibits AID-induced antibody gene conversion
- PMID: 17355182
- PMCID: PMC1820612
- DOI: 10.1371/journal.pbio.0050080
DNA-dependent protein kinase inhibits AID-induced antibody gene conversion
Abstract
Affinity maturation and class switching of antibodies requires activation-induced cytidine deaminase (AID)-dependent hypermutation of Ig V(D)J rearrangements and Ig S regions, respectively, in activated B cells. AID deaminates deoxycytidine bases in Ig genes, converting them into deoxyuridines. In V(D)J regions, subsequent excision of the deaminated bases by uracil-DNA glycosylase, or by mismatch repair, leads to further point mutation or gene conversion, depending on the species. In Ig S regions, nicking at the abasic sites produced by AID and uracil-DNA glycosylases results in staggered double-strand breaks, whose repair by nonhomologous end joining mediates Ig class switching. We have tested whether nonhomologous end joining also plays a role in V(D)J hypermutation using chicken DT40 cells deficient for Ku70 or the DNA-dependent protein kinase catalytic subunit (DNA-PKcs). Inactivation of the Ku70 or DNA-PKcs genes in DT40 cells elevated the rate of AID-induced gene conversion as much as 5-fold. Furthermore, DNA-PKcs-deficiency appeared to reduce point mutation. The data provide strong evidence that double-strand DNA ends capable of recruiting the DNA-dependent protein kinase complex are important intermediates in Ig V gene conversion.
Conflict of interest statement
Figures
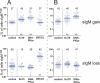
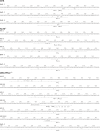
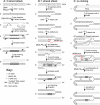
Similar articles
-
Immunoglobulin gene conversion in chicken DT40 cells largely proceeds through an abasic site intermediate generated by excision of the uracil produced by AID-mediated deoxycytidine deamination.Eur J Immunol. 2004 Feb;34(2):504-8. doi: 10.1002/eji.200324631. Eur J Immunol. 2004. PMID: 14768055
-
Brca1 in immunoglobulin gene conversion and somatic hypermutation.DNA Repair (Amst). 2008 Feb 1;7(2):253-66. doi: 10.1016/j.dnarep.2007.10.002. Epub 2007 Nov 26. DNA Repair (Amst). 2008. PMID: 18036997 Free PMC article.
-
AID- and Ung-dependent generation of staggered double-strand DNA breaks in immunoglobulin class switch DNA recombination: a post-cleavage role for AID.Mol Immunol. 2008 Nov;46(1):45-61. doi: 10.1016/j.molimm.2008.07.003. Epub 2008 Aug 28. Mol Immunol. 2008. PMID: 18760480 Free PMC article.
-
Activation-induced cytidine deaminase-mediated hypermutation in the DT40 cell line.Philos Trans R Soc Lond B Biol Sci. 2009 Mar 12;364(1517):639-44. doi: 10.1098/rstb.2008.0202. Philos Trans R Soc Lond B Biol Sci. 2009. PMID: 19008193 Free PMC article. Review.
-
Molecular mechanisms of antibody somatic hypermutation.Annu Rev Biochem. 2007;76:1-22. doi: 10.1146/annurev.biochem.76.061705.090740. Annu Rev Biochem. 2007. PMID: 17328676 Review.
Cited by
-
Checkpoint kinase 2 is required for efficient immunoglobulin diversification.Cell Cycle. 2014;13(23):3659-69. doi: 10.4161/15384101.2014.964112. Cell Cycle. 2014. PMID: 25483076 Free PMC article.
-
SAMHD1 enhances immunoglobulin hypermutation by promoting transversion mutation.Proc Natl Acad Sci U S A. 2018 May 8;115(19):4921-4926. doi: 10.1073/pnas.1719771115. Epub 2018 Apr 18. Proc Natl Acad Sci U S A. 2018. PMID: 29669924 Free PMC article.
-
Incorporation of dUTP does not mediate mutation of A:T base pairs in Ig genes in vivo.Nucleic Acids Res. 2010 Dec;38(22):8120-30. doi: 10.1093/nar/gkq682. Epub 2010 Aug 12. Nucleic Acids Res. 2010. PMID: 20705648 Free PMC article.
-
Competition between PARP-1 and Ku70 control the decision between high-fidelity and mutagenic DNA repair.DNA Repair (Amst). 2011 Mar 7;10(3):338-43. doi: 10.1016/j.dnarep.2010.12.005. Epub 2011 Jan 20. DNA Repair (Amst). 2011. PMID: 21256093 Free PMC article.
-
Genetic evidence for single-strand lesions initiating Nbs1-dependent homologous recombination in diversification of Ig v in chicken B lymphocytes.PLoS Genet. 2009 Jan;5(1):e1000356. doi: 10.1371/journal.pgen.1000356. Epub 2009 Jan 30. PLoS Genet. 2009. PMID: 19180185 Free PMC article.
References
-
- Rooney S, Chaudhuri J, Alt FW. The role of the nonhomologous end joining pathway in lymphocyte development. Immunol Rev. 2004;200:115–131. - PubMed
-
- Jolly CJ, Williams GT, Köhler J, Neuberger MS. The contribution of somatic hypermutation to the diversity of serum immunoglobulin increases dramatically with age. Immunity. 2000;13:409–417. - PubMed
-
- Muramatsu M, Kinoshita K, Fagarasan S, Yamada S, Shinkai Y, et al. Class switch recombination and hypermutation require activation-induced cytidine deaminase (AID), a potential RNA-editing enzyme. Cell. 2000;102:553–563. - PubMed
-
- Revy P, Muto T, Levy Y, Geissmann F, Plebani A, et al. Activation-induced cytidine deaminase (AID) deficiency causes the autosomal recessive form of the Hyper-IgM syndrome (HIGM2) Cell. 2000;102:565–575. - PubMed
-
- Rada C, Gonzalez FA, Jarvis JM, Milstein C. The 5′ boundary of somatic hypermutation in a V kappa gene is in the leader intron. Euro J Immunol. 1994;24:1453–1457. - PubMed
Publication types
MeSH terms
Substances
LinkOut - more resources
Full Text Sources
Molecular Biology Databases