Novel cell death program leads to neutrophil extracellular traps
- PMID: 17210947
- PMCID: PMC2063942
- DOI: 10.1083/jcb.200606027
Novel cell death program leads to neutrophil extracellular traps
Abstract
Neutrophil extracellular traps (NETs) are extracellular structures composed of chromatin and granule proteins that bind and kill microorganisms. We show that upon stimulation, the nuclei of neutrophils lose their shape, and the eu- and heterochromatin homogenize. Later, the nuclear envelope and the granule membranes disintegrate, allowing the mixing of NET components. Finally, the NETs are released as the cell membrane breaks. This cell death process is distinct from apoptosis and necrosis and depends on the generation of reactive oxygen species (ROS) by NADPH oxidase. Patients with chronic granulomatous disease carry mutations in NADPH oxidase and cannot activate this cell-death pathway or make NETs. This novel ROS-dependent death allows neutrophils to fulfill their antimicrobial function, even beyond their lifespan.
Figures
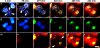
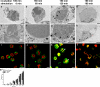
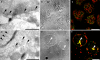
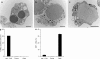
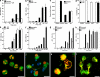
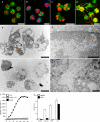
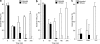
Similar articles
-
Neutrophil extracellular traps: how to generate and visualize them.J Vis Exp. 2010 Feb 24;(36):1724. doi: 10.3791/1724. J Vis Exp. 2010. PMID: 20182410 Free PMC article.
-
Neutrophil extracellular trap in human diseases.Adv Exp Med Biol. 2013;756:1-8. doi: 10.1007/978-94-007-4549-0_1. Adv Exp Med Biol. 2013. PMID: 22836612
-
Uric acid induces NADPH oxidase-independent neutrophil extracellular trap formation.Biochem Biophys Res Commun. 2014 Jan 10;443(2):556-61. doi: 10.1016/j.bbrc.2013.12.007. Epub 2013 Dec 8. Biochem Biophys Res Commun. 2014. PMID: 24326071
-
Neutrophil extracellular traps as a new paradigm in innate immunity: friend or foe?Periodontol 2000. 2013 Oct;63(1):165-97. doi: 10.1111/prd.12025. Periodontol 2000. 2013. PMID: 23931060 Review.
-
[NET and NETosis--new phenomenon in immunology].Postepy Hig Med Dosw (Online). 2012 Jun 22;66:437-45. doi: 10.5604/17322693.1001178. Postepy Hig Med Dosw (Online). 2012. PMID: 22922143 Review. Polish.
Cited by
-
Impact of Nitric Oxide on Polymorphonuclear Neutrophils' Function.Biomedicines. 2024 Oct 16;12(10):2353. doi: 10.3390/biomedicines12102353. Biomedicines. 2024. PMID: 39457665 Free PMC article.
-
How Do ROS Induce NETosis? Oxidative DNA Damage, DNA Repair, and Chromatin Decondensation.Biomolecules. 2024 Oct 16;14(10):1307. doi: 10.3390/biom14101307. Biomolecules. 2024. PMID: 39456240 Free PMC article. Review.
-
Mitophagy in Cell Death Regulation: Insights into Mechanisms and Disease Implications.Biomolecules. 2024 Oct 9;14(10):1270. doi: 10.3390/biom14101270. Biomolecules. 2024. PMID: 39456203 Free PMC article. Review.
-
Dynamic roles of neutrophil extracellular traps in cancer cell adhesion and activation of Notch 1-mediated epithelial-to-mesenchymal transition in EGFR-driven lung cancer cells.Front Immunol. 2024 Oct 4;15:1470620. doi: 10.3389/fimmu.2024.1470620. eCollection 2024. Front Immunol. 2024. PMID: 39430758 Free PMC article.
-
GATA1-deficient human pluripotent stem cells generate neutrophils with improved antifungal immunity that is mediated by the integrin CD18.bioRxiv [Preprint]. 2024 Oct 11:2024.10.11.617742. doi: 10.1101/2024.10.11.617742. bioRxiv. 2024. PMID: 39416161 Free PMC article. Preprint.
References
-
- Aga, E., D.M. Katschinski, G. van Zandbergen, H. Laufs, B. Hansen, K. Muller, W. Solbach, and T. Laskay. 2002. Inhibition of the spontaneous apoptosis of neutrophil granulocytes by the intracellular parasite Leishmania major. J. Immunol. 169:898–905. - PubMed
-
- Beiter, K., F. Wartha, B. Albiger, S. Normark, A. Zychlinsky, and B. Henriques-Normark. 2006. An endonuclease allows Streptococcus pneumoniae to escape from neutrophil extracellular traps. Curr. Biol. 16:401–407. - PubMed
-
- Borregaard, N., and J.B. Cowland. 1997. Granules of the human neutrophilic polymorphonuclear leukocyte. Blood. 89:3503–3521. - PubMed
-
- Brinkmann, V., U. Reichard, C. Goosmann, B. Fauler, Y. Uhlemann, D.S. Weiss, Y. Weinrauch, and A. Zychlinsky. 2004. Neutrophil extracellular traps kill bacteria. Science. 303:1532–1535. - PubMed
-
- Buchanan, J.T., A.J. Simpson, R.K. Aziz, G.Y. Liu, S.A. Kristian, M. Kotb, J. Feramisco, and V. Nizet. 2006. DNase expression allows the pathogen group A Streptococcus to escape killing in neutrophil extracellular traps. Curr. Biol. 16:396–400. - PubMed
Publication types
MeSH terms
Substances
LinkOut - more resources
Full Text Sources
Other Literature Sources