Actin turnover-dependent fast dissociation of capping protein in the dendritic nucleation actin network: evidence of frequent filament severing
- PMID: 17178911
- PMCID: PMC2064704
- DOI: 10.1083/jcb.200604176
Actin turnover-dependent fast dissociation of capping protein in the dendritic nucleation actin network: evidence of frequent filament severing
Abstract
Actin forms the dendritic nucleation network and undergoes rapid polymerization-depolymerization cycles in lamellipodia. To elucidate the mechanism of actin disassembly, we characterized molecular kinetics of the major filament end-binding proteins Arp2/3 complex and capping protein (CP) using single-molecule speckle microscopy. We have determined the dissociation rates of Arp2/3 and CP as 0.048 and 0.58 s(-1), respectively, in lamellipodia of live XTC fibroblasts. This CP dissociation rate is three orders of magnitude faster than in vitro. CP dissociates slower from actin stress fibers than from the lamellipodial actin network, suggesting that CP dissociation correlates with actin filament dynamics. We found that jasplakinolide, an actin depolymerization inhibitor, rapidly blocked the fast CP dissociation in cells. Consistently, the coexpression of LIM kinase prolonged CP speckle lifetime in lamellipodia. These results suggest that cofilin-mediated actin disassembly triggers CP dissociation from actin filaments. We predict that filament severing and end-to-end annealing might take place fairly frequently in the dendritic nucleation actin arrays.
Figures
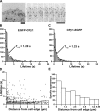
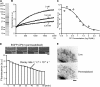
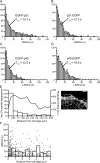
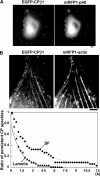
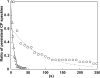
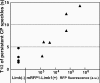
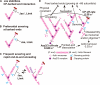
Similar articles
-
Single-molecule speckle analysis of actin filament turnover in lamellipodia.Science. 2002 Feb 8;295(5557):1083-6. doi: 10.1126/science.1067470. Science. 2002. PMID: 11834838
-
Spatial and temporal relationships between actin-filament nucleation, capping, and disassembly.Curr Biol. 2007 Mar 6;17(5):395-406. doi: 10.1016/j.cub.2007.02.012. Curr Biol. 2007. PMID: 17331727 Free PMC article.
-
The Arp2/3 activator WASH controls the fission of endosomes through a large multiprotein complex.Dev Cell. 2009 Nov;17(5):712-23. doi: 10.1016/j.devcel.2009.09.010. Dev Cell. 2009. PMID: 19922875
-
Mechanism of depolymerization and severing of actin filaments and its significance in cytoskeletal dynamics.Int Rev Cytol. 2007;258:1-82. doi: 10.1016/S0074-7696(07)58001-0. Int Rev Cytol. 2007. PMID: 17338919 Review.
-
How do in vitro reconstituted actin-based motility assays provide insight into in vivo behavior?FEBS Lett. 2008 Jun 18;582(14):2086-92. doi: 10.1016/j.febslet.2008.02.065. Epub 2008 Mar 5. FEBS Lett. 2008. PMID: 18328266 Review.
Cited by
-
Internetwork competition for monomers governs actin cytoskeleton organization.Nat Rev Mol Cell Biol. 2016 Dec;17(12):799-810. doi: 10.1038/nrm.2016.106. Epub 2016 Sep 14. Nat Rev Mol Cell Biol. 2016. PMID: 27625321 Free PMC article.
-
V-1 regulates capping protein activity in vivo.Proc Natl Acad Sci U S A. 2016 Oct 25;113(43):E6610-E6619. doi: 10.1073/pnas.1605350113. Epub 2016 Oct 10. Proc Natl Acad Sci U S A. 2016. PMID: 27791032 Free PMC article.
-
Two distinct mechanisms for actin capping protein regulation--steric and allosteric inhibition.PLoS Biol. 2010 Jul 6;8(7):e1000416. doi: 10.1371/journal.pbio.1000416. PLoS Biol. 2010. PMID: 20625546 Free PMC article.
-
VASP is a processive actin polymerase that requires monomeric actin for barbed end association.J Cell Biol. 2010 Nov 1;191(3):571-84. doi: 10.1083/jcb.201003014. J Cell Biol. 2010. PMID: 21041447 Free PMC article.
-
Capping protein increases the rate of actin-based motility by promoting filament nucleation by the Arp2/3 complex.Cell. 2008 May 30;133(5):841-51. doi: 10.1016/j.cell.2008.04.011. Cell. 2008. PMID: 18510928 Free PMC article.
References
-
- Andrianantoandro, E., L. Blanchoin, D. Sept, J.A. McCammon, and T.D. Pollard. 2001. Kinetic mechanism of end-to-end annealing of actin filaments. J. Mol. Biol. 312:721–730. - PubMed
-
- Bamburg, J.R. 1999. Proteins of the ADF/cofilin family: essential regulators of actin dynamics. Annu. Rev. Cell Dev. Biol. 15:185–230. - PubMed
Publication types
MeSH terms
Substances
LinkOut - more resources
Full Text Sources
Other Literature Sources
Miscellaneous