Molecular epidemiology of tuberculosis: current insights
- PMID: 17041139
- PMCID: PMC1592690
- DOI: 10.1128/CMR.00061-05
Molecular epidemiology of tuberculosis: current insights
Abstract
Molecular epidemiologic studies of tuberculosis (TB) have focused largely on utilizing molecular techniques to address short- and long-term epidemiologic questions, such as in outbreak investigations and in assessing the global dissemination of strains, respectively. This is done primarily by examining the extent of genetic diversity of clinical strains of Mycobacterium tuberculosis. When molecular methods are used in conjunction with classical epidemiology, their utility for TB control has been realized. For instance, molecular epidemiologic studies have added much-needed accuracy and precision in describing transmission dynamics, and they have facilitated investigation of previously unresolved issues, such as estimates of recent-versus-reactive disease and the extent of exogenous reinfection. In addition, there is mounting evidence to suggest that specific strains of M. tuberculosis belonging to discrete phylogenetic clusters (lineages) may differ in virulence, pathogenesis, and epidemiologic characteristics, all of which may significantly impact TB control and vaccine development strategies. Here, we review the current methods, concepts, and applications of molecular approaches used to better understand the epidemiology of TB.
Figures
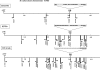
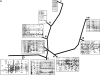
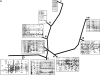
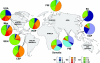
Similar articles
-
Importance of differential identification of Mycobacterium tuberculosis strains for understanding differences in their prevalence, treatment efficacy, and vaccine development.J Microbiol. 2018 May;56(5):300-311. doi: 10.1007/s12275-018-8041-3. Epub 2018 May 2. J Microbiol. 2018. PMID: 29721826 Review.
-
Molecular epidemiology and transmission dynamics of Mycobacterium tuberculosis in Northwest Ethiopia: new phylogenetic lineages found in Northwest Ethiopia.BMC Infect Dis. 2013 Mar 11;13:131. doi: 10.1186/1471-2334-13-131. BMC Infect Dis. 2013. PMID: 23496968 Free PMC article.
-
Drug-Resistant Characteristics, Genetic Diversity, and Transmission Dynamics of Rifampicin-Resistant Mycobacterium tuberculosis in Hunan, China, Revealed by Whole-Genome Sequencing.Microbiol Spectr. 2022 Feb 23;10(1):e0154321. doi: 10.1128/spectrum.01543-21. Epub 2022 Feb 16. Microbiol Spectr. 2022. PMID: 35171016 Free PMC article.
-
[New era in molecular epidemiology of tuberculosis in Japan].Kekkaku. 2006 Nov;81(11):693-707. Kekkaku. 2006. PMID: 17154049 Review. Japanese.
-
Texas tuberculosis research symposium 2018: Research and clinical collaboration within the State of Texas - Moving towards elimination of TB.Tuberculosis (Edinb). 2019 May;116S:S1. doi: 10.1016/j.tube.2019.04.004. Epub 2019 May 16. Tuberculosis (Edinb). 2019. PMID: 31126719 No abstract available.
Cited by
-
Clonality and micro-diversity of a nationwide spreading genotype of Mycobacterium tuberculosis in Japan.PLoS One. 2015 Mar 3;10(3):e0118495. doi: 10.1371/journal.pone.0118495. eCollection 2015. PLoS One. 2015. PMID: 25734518 Free PMC article.
-
Association of diabetes and tuberculosis: impact on treatment and post-treatment outcomes.Thorax. 2013 Mar;68(3):214-20. doi: 10.1136/thoraxjnl-2012-201756. Epub 2012 Dec 18. Thorax. 2013. PMID: 23250998 Free PMC article.
-
Mycobacterial L-forms are found in cord blood: A potential vertical transmission of BCG from vaccinated mothers.Hum Vaccin Immunother. 2016 Oct 2;12(10):2565-2571. doi: 10.1080/21645515.2016.1193658. Epub 2016 Jun 13. Hum Vaccin Immunother. 2016. PMID: 27294392 Free PMC article.
-
Association between vitamin D receptor polymorphisms and haplotypes with pulmonary tuberculosis.Biomed Rep. 2015 Mar;3(2):189-194. doi: 10.3892/br.2014.402. Epub 2014 Dec 15. Biomed Rep. 2015. PMID: 26075071 Free PMC article.
-
Modern lineages of Mycobacterium tuberculosis in Addis Ababa, Ethiopia: implications for the tuberculosis control programe.Afr Health Sci. 2012 Sep;12(3):339-44. doi: 10.4314/ahs.v12i3.15. Afr Health Sci. 2012. PMID: 23382750 Free PMC article.
References
-
- Agerton, T. B., S. E. Valway, R. J. Blinkhorn, K. L. Shilkret, R. Reves, W. W. Schluter, B. Gore, C. J. Pozsik, B. B. Plikaytis, C. Woodley, and I. M. Onorato. 1999. Spread of strain W, a highly drug-resistant strain of Mycobacterium tuberculosis, across the United States. Clin. Infect. Dis. 29:85-95. - PubMed
-
- Alland, D., G. E. Kalkut, A. R. Moss, R. A. McAdam, J. A. Hahn, W. Bosworth, E. Drucker, and B. R. Bloom. 1994. Transmission of tuberculosis in New York City. An analysis by DNA fingerprinting and conventional epidemiologic methods. N. Engl. J. Med. 330:1710-1716. - PubMed
-
- Alland, D., T. S. Whittam, M. B. Murray, M. D. Cave, M. H. Hazbon, K. Dix, M. Kokoris, A. Duesterhoeft, J. A. Eisen, C. M. Fraser, and R. D. Fleischmann. 2003. Modeling bacterial evolution with comparative-genome-based marker systems: application to Mycobacterium tuberculosis evolution and pathogenesis. J. Bacteriol. 185:3392-3399. - PMC - PubMed
-
- American Thoracic Society. 2000. Targeted tuberculin testing and treatment of latent tuberculosis infection. Am. J. Respir. Crit. Care Med. 161:S221-S247. - PubMed
-
- Anderson, R. M., and R. M. May. 1991. Infectious diseases of humans: dynamics and control. Oxford University Press, Oxford, United Kingdom.
Publication types
MeSH terms
Substances
LinkOut - more resources
Full Text Sources
Other Literature Sources
Medical