Mammalian CLASP1 and CLASP2 cooperate to ensure mitotic fidelity by regulating spindle and kinetochore function
- PMID: 16914514
- PMCID: PMC1635371
- DOI: 10.1091/mbc.e06-07-0579
Mammalian CLASP1 and CLASP2 cooperate to ensure mitotic fidelity by regulating spindle and kinetochore function
Abstract
CLASPs are widely conserved microtubule plus-end-tracking proteins with essential roles in the local regulation of microtubule dynamics. In yeast, Drosophila, and Xenopus, a single CLASP orthologue is present, which is required for mitotic spindle assembly by regulating microtubule dynamics at the kinetochore. In mammals, however, only CLASP1 has been directly implicated in cell division, despite the existence of a second paralogue, CLASP2, whose mitotic roles remain unknown. Here, we show that CLASP2 localization at kinetochores, centrosomes, and spindle throughout mitosis is remarkably similar to CLASP1, both showing fast microtubule-independent turnover rates. Strikingly, primary fibroblasts from Clasp2 knockout mice show numerous spindle and chromosome segregation defects that can be partially rescued by ectopic expression of Clasp1 or Clasp2. Moreover, chromosome segregation rates during anaphase A and B are slower in Clasp2 knockout cells, which is consistent with a role of CLASP2 in the regulation of kinetochore and spindle function. Noteworthy, cell viability/proliferation and spindle checkpoint function were not impaired in Clasp2 knockout cells, but the fidelity of mitosis was strongly compromised, leading to severe chromosomal instability in adult cells. Together, our data support that the partial redundancy of CLASPs during mitosis acts as a possible mechanism to prevent aneuploidy in mammals.
Figures
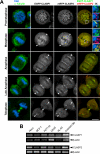
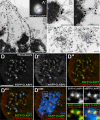
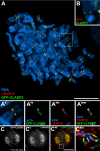
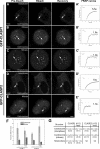
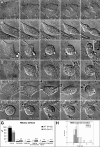
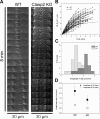
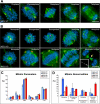
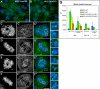
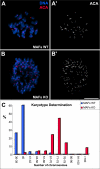
Similar articles
-
CLASP2 binding to curved microtubule tips promotes flux and stabilizes kinetochore attachments.J Cell Biol. 2020 Feb 3;219(2):e201905080. doi: 10.1083/jcb.201905080. J Cell Biol. 2020. PMID: 31757788 Free PMC article.
-
Motor-independent targeting of CLASPs to kinetochores by CENP-E promotes microtubule turnover and poleward flux.Curr Biol. 2009 Sep 29;19(18):1566-72. doi: 10.1016/j.cub.2009.07.059. Epub 2009 Sep 3. Curr Biol. 2009. PMID: 19733075 Free PMC article.
-
Mammalian CLASPs are required for mitotic spindle organization and kinetochore alignment.Genes Cells. 2006 Aug;11(8):845-57. doi: 10.1111/j.1365-2443.2006.00990.x. Genes Cells. 2006. PMID: 16866869
-
Factors that Control Mitotic Spindle Dynamics.Adv Exp Med Biol. 2017;925:89-101. doi: 10.1007/5584_2016_74. Adv Exp Med Biol. 2017. PMID: 27722958 Review.
-
Merotelic kinetochores in mammalian tissue cells.Philos Trans R Soc Lond B Biol Sci. 2005 Mar 29;360(1455):553-68. doi: 10.1098/rstb.2004.1610. Philos Trans R Soc Lond B Biol Sci. 2005. PMID: 15897180 Free PMC article. Review.
Cited by
-
Stabilization of overlapping microtubules by fission yeast CLASP.Dev Cell. 2007 Dec;13(6):812-27. doi: 10.1016/j.devcel.2007.10.015. Dev Cell. 2007. PMID: 18061564 Free PMC article.
-
Chromosome Segregation Is Biased by Kinetochore Size.Curr Biol. 2018 May 7;28(9):1344-1356.e5. doi: 10.1016/j.cub.2018.03.023. Epub 2018 Apr 26. Curr Biol. 2018. PMID: 29706521 Free PMC article.
-
Clasp2 ensures mitotic fidelity and prevents differentiation of epidermal keratinocytes.J Cell Sci. 2017 Feb 15;130(4):683-688. doi: 10.1242/jcs.194787. Epub 2017 Jan 9. J Cell Sci. 2017. PMID: 28069833 Free PMC article.
-
CLASPs function redundantly to regulate astral microtubules in the C. elegans embryo.Dev Biol. 2012 Aug 15;368(2):242-54. doi: 10.1016/j.ydbio.2012.05.016. Epub 2012 May 19. Dev Biol. 2012. PMID: 22613359 Free PMC article.
-
Organelle Inheritance Control of Mitotic Entry and Progression: Implications for Tissue Homeostasis and Disease.Front Cell Dev Biol. 2019 Jul 23;7:133. doi: 10.3389/fcell.2019.00133. eCollection 2019. Front Cell Dev Biol. 2019. PMID: 31396510 Free PMC article. Review.
References
-
- Akhmanova A., Hoogenraad C. C. Microtubule plus-end-tracking proteins: mechanisms and functions. Curr. Opin. Cell Biol. 2005;17:47–54. - PubMed
-
- Akhmanova A., et al. Clasps are CLIP-115 and -170 associating proteins involved in the regional regulation of microtubule dynamics in motile fibroblasts. Cell. 2001;104:923–935. - PubMed
-
- Amin-Hanjani S., Wadsworth P. Inhibition of spindle elongation by taxol. Cell Motil. Cytoskeleton. 1991;20:136–144. - PubMed
-
- Ault J. G., DeMarco A. J., Salmon E. D., Rieder C. L. Studies on the ejection properties of asters: astral microtubule turnover influences the oscillatory behavior and positioning of mono-oriented chromosomes. J. Cell Sci. 1991;99:701–710. - PubMed
Publication types
MeSH terms
Substances
Grants and funding
LinkOut - more resources
Full Text Sources
Molecular Biology Databases