Spontaneous rhythmic field potentials of isolated mouse hippocampal-subicular-entorhinal cortices in vitro
- PMID: 16887877
- PMCID: PMC1890361
- DOI: 10.1113/jphysiol.2006.114918
Spontaneous rhythmic field potentials of isolated mouse hippocampal-subicular-entorhinal cortices in vitro
Abstract
The rodent hippocampal circuit is capable of exhibiting in vitro spontaneous rhythmic field potentials (SRFPs) of 1-4 Hz that originate from the CA3 area and spread to the CA1 area. These SRFPs are largely correlated with GABA-A IPSPs in pyramidal neurons and repetitive discharges in inhibitory interneurons. As such, their generation is thought to result from cooperative network activities involving both pyramidal neurons and GABAergic interneurons. Considering that the hippocampus, subiculum and entorhinal cortex function as an integrated system crucial for memory and cognition, it is of interest to know whether similar SRFPs occur in hippocampal output structures (that is, the subiculum and entorhinal cortex), and if so, to understand the cellular basis of these subicular and entorhinal SRFPs as well as their temporal relation to hippocampal SRFPs. We explored these issues in the present study using thick hippocampal-subicular-entorhinal cortical slices prepared from adult mice. SRFPs were found to spread from the CA1 area to the subicular and entorhinal cortical areas. Subicular and entorhinal cortical SRFPs were correlated with mixed IPSPs/EPSPs in local pyramidal neurons, and their generation was dependent upon the activities of GABA-A and AMPA glutamate receptors. In addition, the isolated subicular circuit could elicit SRFPs independent of CA3 inputs. We hypothesize that the SRFPs represent a basal oscillatory activity of the hippocampal-subicular-entorhinal cortices and that the subiculum functions as both a relay and an amplifier, spreading the SRFPs from the hippocampus to the entorhinal cortex.
Figures
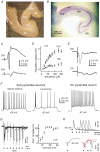
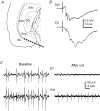
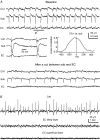
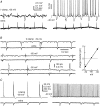
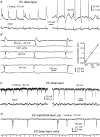
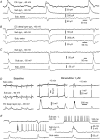
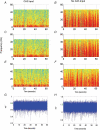
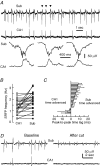
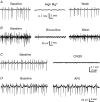
Similar articles
-
Postnatal development of intrinsic GABAergic rhythms in mouse hippocampus.Neuroscience. 2005;134(1):107-20. doi: 10.1016/j.neuroscience.2005.04.019. Neuroscience. 2005. PMID: 15961234
-
Hippocampus as comparator: role of the two input and two output systems of the hippocampus in selection and registration of information.Hippocampus. 2001;11(5):578-98. doi: 10.1002/hipo.1073. Hippocampus. 2001. PMID: 11732710 Review.
-
Preservation of topography in the connections between the subiculum, field CA1, and the entorhinal cortex in rats.J Comp Neurol. 1995 Mar 13;353(3):379-90. doi: 10.1002/cne.903530306. J Comp Neurol. 1995. PMID: 7538515
-
Place cells and place recognition maintained by direct entorhinal-hippocampal circuitry.Science. 2002 Jun 21;296(5576):2243-6. doi: 10.1126/science.1071089. Science. 2002. PMID: 12077421
-
[Neural mechanism underlying generation of synchronous oscillations in hippocampal network].Brain Nerve. 2008 Jul;60(7):755-62. Brain Nerve. 2008. PMID: 18646615 Review. Japanese.
Cited by
-
Electrographic Features of Spontaneous Recurrent Seizures in a Mouse Model of Extended Hippocampal Kindling.Cereb Cortex Commun. 2021 Jan 22;2(1):tgab004. doi: 10.1093/texcom/tgab004. eCollection 2021. Cereb Cortex Commun. 2021. PMID: 34296153 Free PMC article.
-
Fast and slow γ rhythms are intrinsically and independently generated in the subiculum.J Neurosci. 2011 Aug 24;31(34):12104-17. doi: 10.1523/JNEUROSCI.1370-11.2011. J Neurosci. 2011. PMID: 21865453 Free PMC article.
-
Recruitment of oriens-lacunosum-moleculare interneurons during hippocampal ripples.Proc Natl Acad Sci U S A. 2013 Mar 12;110(11):4398-403. doi: 10.1073/pnas.1215496110. Epub 2013 Feb 25. Proc Natl Acad Sci U S A. 2013. PMID: 23440221 Free PMC article.
-
Hippocampal sharp wave-ripple: A cognitive biomarker for episodic memory and planning.Hippocampus. 2015 Oct;25(10):1073-188. doi: 10.1002/hipo.22488. Hippocampus. 2015. PMID: 26135716 Free PMC article. Review.
-
Inhibitory networks of fast-spiking interneurons generate slow population activities due to excitatory fluctuations and network multistability.J Neurosci. 2012 Jul 18;32(29):9931-46. doi: 10.1523/JNEUROSCI.5446-11.2012. J Neurosci. 2012. PMID: 22815508 Free PMC article.
References
-
- Akay M. New York: IEEE Press; 1997. Time-frequency and wavelets in biomedical signal processing.
-
- Amaral DG, Witter MP. The three-dimensional organization of the hippocampal formation: a review of anatomical data. Neuroscience. 1989;31:571–591. - PubMed
-
- Anderson MI, O'Mara SM. Analysis of recordings of single-unit firing and population activity in the dorsal subiculum of unrestrained, freely moving rats. J Neurophysiol. 2003;90:655–665. - PubMed
-
- Anderton BH, Callahan L, Coleman P, Davies P, Flood D, Jicha GA, Ohm T, Weaver C. Dendritic changes in Alzheimer's disease and factors that may underlie these changes. Prog Neurobiol. 1998;55:595–609. - PubMed
Publication types
MeSH terms
Substances
LinkOut - more resources
Full Text Sources
Research Materials
Miscellaneous