Dual effects of plant steroidal alkaloids on Saccharomyces cerevisiae
- PMID: 16870766
- PMCID: PMC1538658
- DOI: 10.1128/AAC.00289-06
Dual effects of plant steroidal alkaloids on Saccharomyces cerevisiae
Abstract
Many plant species accumulate sterols and triterpenes as antimicrobial glycosides. These secondary metabolites (saponins) provide built-in chemical protection against pest and pathogen attack and can also influence induced defense responses. In addition, they have a variety of important pharmacological properties, including anticancer activity. The biological mechanisms underpinning the varied and diverse effects of saponins on microbes, plants, and animals are only poorly understood despite the ecological and pharmaceutical importance of this major class of plant secondary metabolites. Here we have exploited budding yeast (Saccharomyces cerevisiae) to investigate the effects of saponins on eukaryotic cells. The tomato steroidal glycoalkaloid alpha-tomatine has antifungal activity towards yeast, and this activity is associated with membrane permeabilization. Removal of a single sugar from the tetrasaccharide chain of alpha-tomatine results in a substantial reduction in antimicrobial activity. Surprisingly, the complete loss of sugars leads to enhanced antifungal activity. Experiments with alpha-tomatine and its aglycone tomatidine indicate that the mode of action of tomatidine towards yeast is distinct from that of alpha-tomatine and does not involve membrane permeabilization. Investigation of the effects of tomatidine on yeast by gene expression and sterol analysis indicate that tomatidine inhibits ergosterol biosynthesis. Tomatidine-treated cells accumulate zymosterol rather than ergosterol, which is consistent with inhibition of the sterol C(24) methyltransferase Erg6p. However, erg6 and erg3 mutants (but not erg2 mutants) have enhanced resistance to tomatidine, suggesting a complex interaction of erg mutations, sterol content, and tomatidine resistance.
Figures
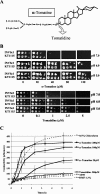
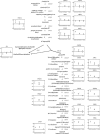
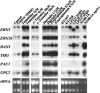
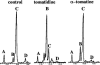
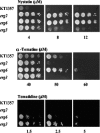
Similar articles
-
Identification and Mode of Action of a Plant Natural Product Targeting Human Fungal Pathogens.Antimicrob Agents Chemother. 2017 Aug 24;61(9):e00829-17. doi: 10.1128/AAC.00829-17. Print 2017 Sep. Antimicrob Agents Chemother. 2017. PMID: 28674054 Free PMC article.
-
Tomatidine promotes the inhibition of 24-alkylated sterol biosynthesis and mitochondrial dysfunction in Leishmania amazonensis promastigotes.Parasitology. 2012 Sep;139(10):1253-65. doi: 10.1017/S0031182012000522. Epub 2012 May 1. Parasitology. 2012. PMID: 22716777
-
Effect of alpha-tomatine and tomatidine on membrane potential of frog embryos and active transport of ions in frog skin.Food Chem Toxicol. 1997 Jul;35(7):639-46. doi: 10.1016/s0278-6915(97)00038-0. Food Chem Toxicol. 1997. PMID: 9301646
-
The steroidal alkaloids α-tomatine and tomatidine: Panorama of their mode of action and pharmacological properties.Steroids. 2021 Dec;176:108933. doi: 10.1016/j.steroids.2021.108933. Epub 2021 Oct 23. Steroids. 2021. PMID: 34695457 Review.
-
Therapeutic Potential of Steroidal Alkaloids in Cancer and Other Diseases.Med Res Rev. 2016 Jan;36(1):119-43. doi: 10.1002/med.21346. Epub 2015 Mar 27. Med Res Rev. 2016. PMID: 25820039 Review.
Cited by
-
The Biological Activity of Natural Alkaloids against Herbivores, Cancerous Cells and Pathogens.Toxins (Basel). 2019 Nov 11;11(11):656. doi: 10.3390/toxins11110656. Toxins (Basel). 2019. PMID: 31717922 Free PMC article. Review.
-
Diversity in Chemical Structures and Biological Properties of Plant Alkaloids.Molecules. 2021 Jun 3;26(11):3374. doi: 10.3390/molecules26113374. Molecules. 2021. PMID: 34204857 Free PMC article. Review.
-
Anti-Candida albicans Effects and Mechanisms of Theasaponin E1 and Assamsaponin A.Int J Mol Sci. 2023 May 27;24(11):9350. doi: 10.3390/ijms24119350. Int J Mol Sci. 2023. PMID: 37298302 Free PMC article.
-
A novel flow cytometric HTS assay reveals functional modulators of ATP binding cassette transporter ABCB6.PLoS One. 2012;7(7):e40005. doi: 10.1371/journal.pone.0040005. Epub 2012 Jul 10. PLoS One. 2012. PMID: 22808084 Free PMC article.
-
Characterization of plant-derived saponin natural products against Candida albicans.ACS Chem Biol. 2010 Mar 19;5(3):321-32. doi: 10.1021/cb900243b. ACS Chem Biol. 2010. PMID: 20099897 Free PMC article.
References
-
- Agarwal, A. K., P. D. Rogers, S. R. Baerson, M. R. Jacob, K. S. Barker, J. D. Cleary, L. A. Walker, D. G. Nagle, and A. M. Clark. 2003. Genome-wide expression profiling of the response to polyene, pyrimidine, azole, and echinocandin antifungal agents in Saccharomyces cerevisiae. J. Biol. Chem. 278:34998-35015. - PubMed
-
- Arthington, B. A., L. G. Bennett, P. L. Skatrud, C. J. Guynn, R. J. Barbuch, C. E. Ulbright, and M. Bard. 1991. Cloning, disruption and sequence of the gene encoding yeast C-5 sterol desaturase. Gene 102:39-44. - PubMed
-
- Ashman, W. H., R. J. Barbuch, C. E. Ulbright, H. W. Jarrett, and M. Bard. 1991. Cloning and disruption of the yeast C-8 sterol isomerase gene. Lipids 26:628-632. - PubMed
-
- Bard, M., R. A. Woods, D. H. Barton, J. E. Corrie, and D. A. Widdowson. 1977. Sterol mutants of Saccharomyces cerevisiae: chromatographic analyses. Lipids 12:645-654. - PubMed
Publication types
MeSH terms
Substances
LinkOut - more resources
Full Text Sources
Other Literature Sources
Molecular Biology Databases