Hepatocyte nuclear factor-4alpha contributes to carbohydrate-induced transcriptional activation of hepatic fatty acid synthase
- PMID: 16800817
- PMCID: PMC1609920
- DOI: 10.1042/BJ20060659
Hepatocyte nuclear factor-4alpha contributes to carbohydrate-induced transcriptional activation of hepatic fatty acid synthase
Abstract
Refeeding a carbohydrate-rich meal after a fast produces a co-ordinated induction of key glycolytic and lipogenic genes in the liver. The transcriptional response is mediated by insulin and increased glucose oxidation, and both signals are necessary for optimal induction of FAS (fatty acid synthase). The glucose-regulated component of FAS promoter activation is mediated in part by ChREBP [ChoRE (carbohydrate response element)-binding protein], which binds to a ChoRE between -7300 and -7000 base-pairs in a carbohydrate-dependent manner. Using in vivo footprinting with nuclei from fasted and refed rats, we identify an imperfect DR-1 (direct repeat-1) element between -7110 and -7090 bp that is protected upon carbohydrate refeeding. Electrophoretic mobility-shift assays establish that this DR-1 element binds HNF-4alpha (hepatocyte nuclear factor 4alpha), and chromatin immunoprecipitation establishes that HNF-4alpha binding to this site is increased approx. 3-fold by glucose refeeding. HNF-4alpha transactivates reporter constructs containing the distal FAS promoter in a DR-1-dependent manner, and this DR-1 is required for full glucose induction of the FAS promoter in primary hepatocytes. In addition, a 3-fold knockdown of hepatocyte HNF-4alpha by small interfering RNA produces a corresponding decrease in FAS gene induction by glucose. Co-immunoprecipitation experiments demonstrate a physical interaction between HNF-4alpha and ChREBP in primary hepatocytes, further supporting an important complementary role for HNF-4alpha in glucose-induced activation of FAS transcription. Taken together, these observations establish for the first time that HNF-4alpha functions in vivo through a DR-1 element in the distal FAS promoter to enhance gene transcription following refeeding of glucose to fasted rats. The findings support the broader view that HNF-4alpha is an integral component of the hepatic nutrient sensing system that co-ordinates transcriptional responses to transitions between nutritional states.
Figures
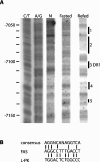
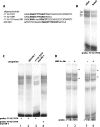
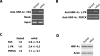
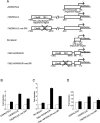
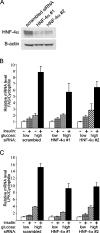
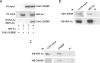
Similar articles
-
Identification of HNF-4α as a key transcription factor to promote ChREBP expression in response to glucose.Sci Rep. 2016 Mar 31;6:23944. doi: 10.1038/srep23944. Sci Rep. 2016. PMID: 27029511 Free PMC article.
-
Regulation of rat hepatic L-pyruvate kinase promoter composition and activity by glucose, n-3 polyunsaturated fatty acids, and peroxisome proliferator-activated receptor-alpha agonist.J Biol Chem. 2006 Jul 7;281(27):18351-62. doi: 10.1074/jbc.M601277200. Epub 2006 Apr 27. J Biol Chem. 2006. PMID: 16644726 Free PMC article.
-
Regulation of glucose-6-phosphatase gene expression in cultured hepatocytes and H4IIE cells by short-chain fatty acids: role of hepatic nuclear factor-4alpha.J Biol Chem. 2003 Oct 17;278(42):40694-701. doi: 10.1074/jbc.M303182200. Epub 2003 Aug 11. J Biol Chem. 2003. PMID: 12915406
-
Involvement of a unique carbohydrate-responsive factor in the glucose regulation of rat liver fatty-acid synthase gene transcription.J Biol Chem. 2001 Jun 15;276(24):21969-75. doi: 10.1074/jbc.M100461200. Epub 2001 Mar 28. J Biol Chem. 2001. PMID: 11279238
-
Roles of Vitamin A Metabolism in the Development of Hepatic Insulin Resistance.ISRN Hepatol. 2013 Sep 30;2013:534972. doi: 10.1155/2013/534972. eCollection 2013. ISRN Hepatol. 2013. PMID: 27335827 Free PMC article. Review.
Cited by
-
From Food to Genes: Transcriptional Regulation of Metabolism by Lipids and Carbohydrates.Nutrients. 2021 Apr 30;13(5):1513. doi: 10.3390/nu13051513. Nutrients. 2021. PMID: 33946267 Free PMC article. Review.
-
Regulation of Carbohydrate Energy Metabolism in Drosophila melanogaster.Genetics. 2017 Dec;207(4):1231-1253. doi: 10.1534/genetics.117.199885. Genetics. 2017. PMID: 29203701 Free PMC article. Review.
-
Modification of a Constitutive to Glucose-Responsive Liver-Specific Promoter Resulted in Increased Efficacy of Adeno-Associated Virus Serotype 8-Insulin Gene Therapy of Diabetic Mice.Cells. 2020 Nov 13;9(11):2474. doi: 10.3390/cells9112474. Cells. 2020. PMID: 33202992 Free PMC article.
-
Hnf4α Is Involved in LC-PUFA Biosynthesis by Up-Regulating Gene Transcription of Elongase in Marine Teleost Siganus canaliculatus.Int J Mol Sci. 2018 Oct 16;19(10):3193. doi: 10.3390/ijms19103193. Int J Mol Sci. 2018. PMID: 30332813 Free PMC article.
-
Why Is Apolipoprotein CIII Emerging as a Novel Therapeutic Target to Reduce the Burden of Cardiovascular Disease?Curr Atheroscler Rep. 2016 Oct;18(10):59. doi: 10.1007/s11883-016-0614-1. Curr Atheroscler Rep. 2016. PMID: 27613744 Free PMC article. Review.
References
-
- Clarke S. D., Armstrong M. K., Jump D. B. Dietary polyunsaturated fats uniquely suppress rat liver fatty acid synthase and S14 mRNA content. J. Nutr. 1990;120:225–231. - PubMed
-
- Paulauskis J. D., Sul H. S. Hormonal regulation of mouse fatty acid synthase gene transcription in liver. J. Biol. Chem. 1989;264:574–577. - PubMed
-
- Armstrong M. K., Blake W. L., Clarke S. D. Arachidonic acid suppression of fatty acid synthase gene expression in cultured rat hepatocytes. Biochem. Biophys. Res. Commun. 1991;177:1056–1061. - PubMed
-
- Rangan V. S., Oskouian B., Smith S. Identification of an inverted CCAAT box motif in the fatty-acid synthase gene as an essential element for modification of transcriptional regulation by cAMP. J. Biol. Chem. 1996;271:2307–2312. - PubMed
Publication types
MeSH terms
Substances
Grants and funding
LinkOut - more resources
Full Text Sources
Molecular Biology Databases
Research Materials
Miscellaneous