The malarial host-targeting signal is conserved in the Irish potato famine pathogen
- PMID: 16733545
- PMCID: PMC1464399
- DOI: 10.1371/journal.ppat.0020050
The malarial host-targeting signal is conserved in the Irish potato famine pathogen
Abstract
Animal and plant eukaryotic pathogens, such as the human malaria parasite Plasmodium falciparum and the potato late blight agent Phytophthora infestans, are widely divergent eukaryotic microbes. Yet they both produce secretory virulence and pathogenic proteins that alter host cell functions. In P. falciparum, export of parasite proteins to the host erythrocyte is mediated by leader sequences shown to contain a host-targeting (HT) motif centered on an RxLx (E, D, or Q) core: this motif appears to signify a major pathogenic export pathway with hundreds of putative effectors. Here we show that a secretory protein of P. infestans, which is perceived by plant disease resistance proteins and induces hypersensitive plant cell death, contains a leader sequence that is equivalent to the Plasmodium HT-leader in its ability to export fusion of green fluorescent protein (GFP) from the P. falciparum parasite to the host erythrocyte. This export is dependent on an RxLR sequence conserved in P. infestans leaders, as well as in leaders of all ten secretory oomycete proteins shown to function inside plant cells. The RxLR motif is also detected in hundreds of secretory proteins of P. infestans, Phytophthora sojae, and Phytophthora ramorum and has high value in predicting host-targeted leaders. A consensus motif further reveals E/D residues enriched within approximately 25 amino acids downstream of the RxLR, which are also needed for export. Together the data suggest that in these plant pathogenic oomycetes, a consensus HT motif may reside in an extended sequence of approximately 25-30 amino acids, rather than in a short linear sequence. Evidence is presented that although the consensus is much shorter in P. falciparum, information sufficient for vacuolar export is contained in a region of approximately 30 amino acids, which includes sequences flanking the HT core. Finally, positional conservation between Phytophthora RxLR and P. falciparum RxLx (E, D, Q) is consistent with the idea that the context of their presentation is constrained. These studies provide the first evidence to our knowledge that eukaryotic microbes share equivalent pathogenic HT signals and thus conserved mechanisms to access host cells across plant and animal kingdoms that may present unique targets for prophylaxis across divergent pathogens.
Conflict of interest statement
Figures
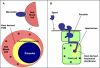
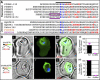
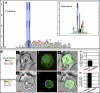
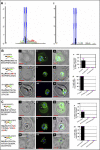
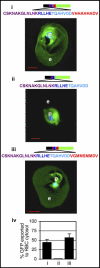
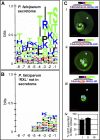
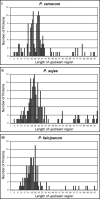
Similar articles
-
Plasmodium falciparum and Hyaloperonospora parasitica effector translocation motifs are functional in Phytophthora infestans.Microbiology (Reading). 2008 Dec;154(Pt 12):3743-3751. doi: 10.1099/mic.0.2008/021964-0. Microbiology (Reading). 2008. PMID: 19047742
-
RXLR-mediated entry of Phytophthora sojae effector Avr1b into soybean cells does not require pathogen-encoded machinery.Plant Cell. 2008 Jul;20(7):1930-47. doi: 10.1105/tpc.107.056093. Epub 2008 Jul 11. Plant Cell. 2008. PMID: 18621946 Free PMC article.
-
The problem of how fungal and oomycete avirulence proteins enter plant cells.Trends Plant Sci. 2006 Feb;11(2):61-3. doi: 10.1016/j.tplants.2005.12.008. Epub 2006 Jan 9. Trends Plant Sci. 2006. PMID: 16406302
-
Common infection strategies of pathogenic eukaryotes.Nat Rev Microbiol. 2006 Dec;4(12):922-31. doi: 10.1038/nrmicro1549. Epub 2006 Nov 6. Nat Rev Microbiol. 2006. PMID: 17088934 Review.
-
Towards understanding the virulence functions of RXLR effectors of the oomycete plant pathogen Phytophthora infestans.J Exp Bot. 2009;60(4):1133-40. doi: 10.1093/jxb/ern353. Epub 2009 Feb 9. J Exp Bot. 2009. PMID: 19204033 Review.
Cited by
-
An Interspecies Comparative Analysis of the Predicted Secretomes of the Necrotrophic Plant Pathogens Sclerotinia sclerotiorum and Botrytis cinerea.PLoS One. 2015 Jun 24;10(6):e0130534. doi: 10.1371/journal.pone.0130534. eCollection 2015. PLoS One. 2015. PMID: 26107498 Free PMC article.
-
Endocytosis in plant-microbe interactions.Protoplasma. 2010 Dec;247(3-4):177-93. doi: 10.1007/s00709-010-0195-8. Epub 2010 Sep 3. Protoplasma. 2010. PMID: 20814704 Review.
-
Host-targeting protein 1 (SpHtp1) from the oomycete Saprolegnia parasitica translocates specifically into fish cells in a tyrosine-O-sulphate-dependent manner.Proc Natl Acad Sci U S A. 2012 Feb 7;109(6):2096-101. doi: 10.1073/pnas.1113775109. Epub 2012 Jan 20. Proc Natl Acad Sci U S A. 2012. PMID: 22308362 Free PMC article.
-
Uptake of the Fusarium Effector Avr2 by Tomato Is Not a Cell Autonomous Event.Front Plant Sci. 2016 Dec 21;7:1915. doi: 10.3389/fpls.2016.01915. eCollection 2016. Front Plant Sci. 2016. PMID: 28066471 Free PMC article.
-
At the Frontier; RXLR Effectors Crossing the Phytophthora-Host Interface.Front Plant Sci. 2011 Nov 1;2:75. doi: 10.3389/fpls.2011.00075. eCollection 2011. Front Plant Sci. 2011. PMID: 22645549 Free PMC article.
References
-
- Lammertyn E, Anne J. Protein secretion in Legionella pneumophila and its relation to virulence. FEMS Microbiol Lett. 2004;238:273–279. - PubMed
-
- Preston GM, Studholme DJ, Caldelari I. Profiling the secretomes of plant pathogenic Proteobacteria. FEMS Microbiol Rev. 2005;29:331–360. - PubMed
-
- Journet L, Hughes KT, Cornelis GR. Type III secretion: A secretory pathway serving both motility and virulence (review) Mol Membr Biol. 2005;22:41–50. - PubMed
-
- Hiller NL, Bhattacharjee S, van Ooij C, Liolios K, Harrison T, et al. A host-targeting signal in virulence proteins reveals a secretome in malarial infection. Science. 2004;306:1934–1937. - PubMed
Publication types
MeSH terms
Substances
Grants and funding
LinkOut - more resources
Full Text Sources
Other Literature Sources