Regulation of Epstein-Barr virus latency type by the chromatin boundary factor CTCF
- PMID: 16731911
- PMCID: PMC1472585
- DOI: 10.1128/JVI.00025-06
Regulation of Epstein-Barr virus latency type by the chromatin boundary factor CTCF
Abstract
Epstein Barr virus (EBV) can establish distinct latency types with different growth-transforming properties. Type I latency and type III latency can be distinguished by the expression of EBNA2, which has been shown to be regulated, in part, by the EBNA1-dependent enhancer activity of the origin of replication (OriP). Here, we report that CTCF, a chromatin boundary factor with well-established enhancer-blocking activity, binds to EBV sequences between the OriP and the RBP-Jkappa response elements of the C promoter (Cp) and regulates transcription levels of EBNA2 mRNA. Using DNA affinity, electrophoretic mobility shift assay, DNase I footprinting, and chromatin immunoprecipitation (ChIP), we found that CTCF binds both in vitro and in vivo to the EBV genome between OriP and Cp, with an approximately 50-bp footprint at EBV coordinates 10515 to 10560. Deletion of this CTCF binding site in a recombinant EBV bacterial artificial chromosome (BAC) increased EBNA2 transcription by 3.5-fold compared to a wild-type EBV BAC. DNA affinity and ChIP showed more CTCF binding at this site in type I latency cell lines (MutuI and KemI) than in type III latency cell lines (LCL3456 and Raji). CTCF protein and mRNA expression levels were higher in type I than type III cell lines. Short interfering RNA depletion of CTCF in type I MutuI cells stimulated EBNA2 mRNA levels, while overexpression of CTCF in type III Raji cells inhibited EBNA2 mRNA levels. These results indicate that increased CTCF can repress EBNA2 transcription. We also show that c-MYC, as well as EBNA2, can stimulate CTCF mRNA levels, suggesting that CTCF levels may contribute to B-cell differentiation as well as EBV latency type determination.
Figures
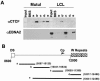
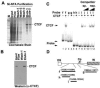
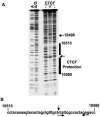
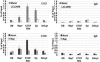
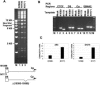
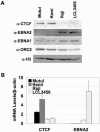
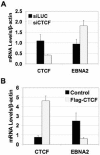
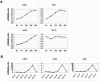
Similar articles
-
Dynamic chromatin boundaries delineate a latency control region of Epstein-Barr virus.J Virol. 2004 Nov;78(22):12308-19. doi: 10.1128/JVI.78.22.12308-12319.2004. J Virol. 2004. PMID: 15507618 Free PMC article.
-
Chromatin profiling of Epstein-Barr virus latency control region.J Virol. 2007 Jun;81(12):6389-401. doi: 10.1128/JVI.02172-06. Epub 2007 Apr 4. J Virol. 2007. PMID: 17409162 Free PMC article.
-
Three restricted forms of Epstein-Barr virus latency counteracting apoptosis in c-myc-expressing Burkitt lymphoma cells.Proc Natl Acad Sci U S A. 2006 Oct 3;103(40):14935-40. doi: 10.1073/pnas.0509988103. Epub 2006 Sep 25. Proc Natl Acad Sci U S A. 2006. PMID: 17001014 Free PMC article.
-
EBNA1 and host factors in Epstein-Barr virus latent DNA replication.Curr Opin Virol. 2012 Dec;2(6):733-9. doi: 10.1016/j.coviro.2012.09.005. Epub 2012 Sep 30. Curr Opin Virol. 2012. PMID: 23031715 Review.
-
Chromatin Structure of Epstein-Barr Virus Latent Episomes.Curr Top Microbiol Immunol. 2015;390(Pt 1):71-102. doi: 10.1007/978-3-319-22822-8_5. Curr Top Microbiol Immunol. 2015. PMID: 26424644 Review.
Cited by
-
Characterization of a new CCCTC-binding factor binding site as a dual regulator of Epstein-Barr virus latent infection.PLoS Pathog. 2023 Jan 25;19(1):e1011078. doi: 10.1371/journal.ppat.1011078. eCollection 2023 Jan. PLoS Pathog. 2023. PMID: 36696451 Free PMC article.
-
Complex Interactions between Cohesin and CTCF in Regulation of Kaposi's Sarcoma-Associated Herpesvirus Lytic Transcription.J Virol. 2020 Jan 6;94(2):e01279-19. doi: 10.1128/JVI.01279-19. Print 2020 Jan 6. J Virol. 2020. PMID: 31666380 Free PMC article.
-
A wide extent of inter-strain diversity in virulent and vaccine strains of alphaherpesviruses.PLoS Pathog. 2011 Oct;7(10):e1002282. doi: 10.1371/journal.ppat.1002282. Epub 2011 Oct 13. PLoS Pathog. 2011. PMID: 22022263 Free PMC article.
-
Epigenetic regulation of EBV persistence and oncogenesis.Semin Cancer Biol. 2014 Jun;26:22-9. doi: 10.1016/j.semcancer.2014.01.003. Epub 2014 Jan 24. Semin Cancer Biol. 2014. PMID: 24468737 Free PMC article. Review.
-
CTCF-dependent chromatin boundary element between the latency-associated transcript and ICP0 promoters in the herpes simplex virus type 1 genome.J Virol. 2007 May;81(10):5192-201. doi: 10.1128/JVI.02447-06. Epub 2007 Jan 31. J Virol. 2007. PMID: 17267480 Free PMC article.
References
-
- Ambinder, R. F., K. D. Robertson, and Q. Tao. 1999. DNA methylation and the Epstein-Barr virus. Semin. Cancer Biol. 9:369-375. - PubMed
-
- Atanasiu, C., L. Lezina, and P. M. Lieberman. 2005. DNA affinity purification of Epstein-Barr virus OriP-binding proteins. Methods Mol. Biol. 292:267-276. - PubMed
-
- Babcock, G. J., D. Hochberg, and A. D. Thorley-Lawson. 2000. The expression pattern of Epstein-Barr virus latent genes in vivo is dependent upon the differentiation stage of the infected B cell. Immunity 13:497-506. - PubMed
Publication types
MeSH terms
Substances
Grants and funding
LinkOut - more resources
Full Text Sources
Miscellaneous