Genetic analysis of synaptotagmin 2 in spontaneous and Ca2+-triggered neurotransmitter release
- PMID: 16642042
- PMCID: PMC1462977
- DOI: 10.1038/sj.emboj.7601103
Genetic analysis of synaptotagmin 2 in spontaneous and Ca2+-triggered neurotransmitter release
Abstract
Synaptotagmin 2 resembles synaptotagmin 1, the Ca2+ sensor for fast neurotransmitter release in forebrain synapses, but little is known about synaptotagmin 2 function. Here, we describe a severely ataxic mouse strain that harbors a single, destabilizing amino-acid substitution (I377N) in synaptotagmin 2. In Calyx of Held synapses, this mutation causes a delay and a decrease in Ca2+-induced but not in hypertonic sucrose-induced release, suggesting that synaptotagmin 2 mediates Ca2+ triggering of evoked release in brainstem synapses. Unexpectedly, we additionally observed in synaptotagmin 2 mutant synapses a dramatic increase in spontaneous release. Synaptotagmin 1-deficient excitatory and inhibitory cortical synapses also displayed a large increase in spontaneous release, demonstrating that this effect was shared among synaptotagmins 1 and 2. Our data suggest that synaptotagmin 1 and 2 perform equivalent functions in the Ca2+ triggering of action potential-induced release and in the restriction of spontaneous release, consistent with a general role of synaptotagmins in controlling 'release slots' for synaptic vesicles at the active zone.
Figures
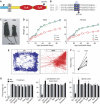
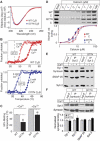
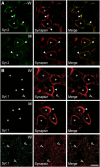
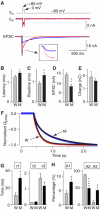
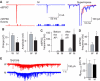
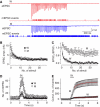
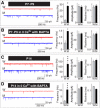
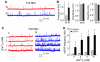
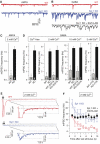
Similar articles
-
Synaptotagmin-2 is essential for survival and contributes to Ca2+ triggering of neurotransmitter release in central and neuromuscular synapses.J Neurosci. 2006 Dec 27;26(52):13493-504. doi: 10.1523/JNEUROSCI.3519-06.2006. J Neurosci. 2006. PMID: 17192432 Free PMC article.
-
Synaptotagmin I: a major Ca2+ sensor for transmitter release at a central synapse.Cell. 1994 Nov 18;79(4):717-27. doi: 10.1016/0092-8674(94)90556-8. Cell. 1994. PMID: 7954835
-
Synaptotagmin IV does not alter excitatory fast synaptic transmission or fusion pore kinetics in mammalian CNS neurons.J Neurosci. 2006 Jan 11;26(2):372-80. doi: 10.1523/JNEUROSCI.3997-05.2006. J Neurosci. 2006. PMID: 16407532 Free PMC article.
-
Vesicle dynamics: how synaptic proteins regulate different modes of neurotransmission.J Neurochem. 2013 Jul;126(2):146-54. doi: 10.1111/jnc.12245. Epub 2013 Apr 23. J Neurochem. 2013. PMID: 23517499 Review.
-
Synaptotagmin in Ca2+ -dependent exocytosis: dynamic action in a flash.Neuron. 2003 May 22;38(4):521-4. doi: 10.1016/s0896-6273(03)00290-3. Neuron. 2003. PMID: 12765604 Review.
Cited by
-
Extracellular vesicles: Roles and applications in drug-induced liver injury.Adv Clin Chem. 2021;102:63-125. doi: 10.1016/bs.acc.2020.08.010. Epub 2020 Oct 1. Adv Clin Chem. 2021. PMID: 34044913 Free PMC article. Review.
-
New recessive mutations in SYT2 causing severe presynaptic congenital myasthenic syndromes.Neurol Genet. 2020 Dec 3;6(6):e534. doi: 10.1212/NXG.0000000000000534. eCollection 2020 Dec. Neurol Genet. 2020. PMID: 33659639 Free PMC article.
-
Disrupted-in-schizophrenia (DISC1) functions presynaptically at glutamatergic synapses.PLoS One. 2012;7(3):e34053. doi: 10.1371/journal.pone.0034053. Epub 2012 Mar 30. PLoS One. 2012. PMID: 22479520 Free PMC article.
-
Spontaneous Vesicle Release Is Not Tightly Coupled to Voltage-Gated Calcium Channel-Mediated Ca2+ Influx and Is Triggered by a Ca2+ Sensor Other Than Synaptotagmin-2 at the Juvenile Mice Calyx of Held Synapses.J Neurosci. 2015 Jul 1;35(26):9632-7. doi: 10.1523/JNEUROSCI.0457-15.2015. J Neurosci. 2015. PMID: 26134646 Free PMC article.
-
A mechanism intrinsic to the vesicle fusion machinery determines fast and slow transmitter release at a large CNS synapse.J Neurosci. 2007 Mar 21;27(12):3198-210. doi: 10.1523/JNEUROSCI.4471-06.2007. J Neurosci. 2007. PMID: 17376981 Free PMC article.
References
-
- DiAntonio A, Schwarz TL (1994) The effect on synaptic physiology of synaptotagmin mutations in Drosophila. Neuron 12: 909–920 - PubMed
MeSH terms
Substances
LinkOut - more resources
Full Text Sources
Molecular Biology Databases
Miscellaneous