Dendritic BC1 RNA in translational control mechanisms
- PMID: 16330711
- PMCID: PMC1828541
- DOI: 10.1083/jcb.200506006
Dendritic BC1 RNA in translational control mechanisms
Erratum in
- J Cell Biol. 2006 Feb 13;172(4):635
Abstract
Translational control at the synapse is thought to be a key determinant of neuronal plasticity. How is such control implemented? We report that small untranslated BC1 RNA is a specific effector of translational control both in vitro and in vivo. BC1 RNA, expressed in neurons and germ cells, inhibits a rate-limiting step in the assembly of translation initiation complexes. A translational repression element is contained within the unique 3' domain of BC1 RNA. Interactions of this domain with eukaryotic initiation factor 4A and poly(A) binding protein mediate repression, indicating that the 3' BC1 domain targets a functional interaction between these factors. In contrast, interactions of BC1 RNA with the fragile X mental retardation protein could not be documented. Thus, BC1 RNA modulates translation-dependent processes in neurons and germs cells by directly interacting with translation initiation factors.
Figures
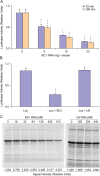
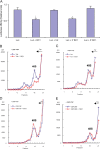
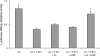
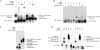
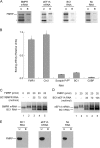
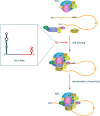
Similar articles
-
Translational control by a small RNA: dendritic BC1 RNA targets the eukaryotic initiation factor 4A helicase mechanism.Mol Cell Biol. 2008 May;28(9):3008-19. doi: 10.1128/MCB.01800-07. Epub 2008 Mar 3. Mol Cell Biol. 2008. PMID: 18316401 Free PMC article.
-
Dendritic BC1 RNA: functional role in regulation of translation initiation.J Neurosci. 2002 Dec 1;22(23):10232-41. doi: 10.1523/JNEUROSCI.22-23-10232.2002. J Neurosci. 2002. PMID: 12451124 Free PMC article.
-
Inhibitory effect of naked neural BC1 RNA or BC200 RNA on eukaryotic in vitro translation systems is reversed by poly(A)-binding protein (PABP).J Mol Biol. 2005 Oct 14;353(1):88-103. doi: 10.1016/j.jmb.2005.07.049. J Mol Biol. 2005. PMID: 16154588
-
Regulation of poly(A)-binding protein through PABP-interacting proteins.Cold Spring Harb Symp Quant Biol. 2006;71:537-43. doi: 10.1101/sqb.2006.71.061. Cold Spring Harb Symp Quant Biol. 2006. PMID: 17381337 Review.
-
[Circular polysomes and translational control].Tanpakushitsu Kakusan Koso. 2001 Sep;46(12):1836-41. Tanpakushitsu Kakusan Koso. 2001. PMID: 11552266 Review. Japanese. No abstract available.
Cited by
-
Identification of Long Non-Coding RNA Expression Profiles and Co-Expression Genes in Thyroid Carcinoma Based on The Cancer Genome Atlas (TCGA) Database.Med Sci Monit. 2019 Dec 19;25:9752-9769. doi: 10.12659/MSM.917845. Med Sci Monit. 2019. PMID: 31856144 Free PMC article.
-
Spatial codes in dendritic BC1 RNA.J Cell Biol. 2006 Nov 6;175(3):427-39. doi: 10.1083/jcb.200607008. Epub 2006 Oct 30. J Cell Biol. 2006. PMID: 17074884 Free PMC article.
-
Regulatory RNAs in brain function and disorders.Brain Res. 2010 Jun 18;1338:36-47. doi: 10.1016/j.brainres.2010.03.042. Epub 2010 Mar 20. Brain Res. 2010. PMID: 20307503 Free PMC article. Review.
-
Negative correlation between expression level and evolutionary rate of long intergenic noncoding RNAs.Genome Biol Evol. 2011;3:1390-404. doi: 10.1093/gbe/evr116. Epub 2011 Nov 9. Genome Biol Evol. 2011. PMID: 22071789 Free PMC article.
-
Translational control by a small RNA: dendritic BC1 RNA targets the eukaryotic initiation factor 4A helicase mechanism.Mol Cell Biol. 2008 May;28(9):3008-19. doi: 10.1128/MCB.01800-07. Epub 2008 Mar 3. Mol Cell Biol. 2008. PMID: 18316401 Free PMC article.
References
-
- Antar, L.N., and G.J. Bassell. 2003. Sunrise at the synapse: the FMRP mRNP shaping the synaptic interface. Neuron. 37:555–558. - PubMed
-
- Audet, R.G., J. Goodchild, and J.D. Richter. 1987. Eukaryotic initiation factor 4A stimulates translation in microinjected Xenopus oocytes. Dev. Biol. 121:58–68. - PubMed
-
- Barciszewski, J., and V.A. Erdmann. 2003. Noncoding RNAs: Molecular Biology and Molecular Medicine. Landes Bioscience, Georgetown, TX. 292 pp.
Publication types
MeSH terms
Substances
Grants and funding
LinkOut - more resources
Full Text Sources
Other Literature Sources