Molecular biology of pseudorabies virus: impact on neurovirology and veterinary medicine
- PMID: 16148307
- PMCID: PMC1197806
- DOI: 10.1128/MMBR.69.3.462-500.2005
Molecular biology of pseudorabies virus: impact on neurovirology and veterinary medicine
Abstract
Pseudorabies virus (PRV) is a herpesvirus of swine, a member of the Alphaherpesvirinae subfamily, and the etiological agent of Aujeszky's disease. This review describes the contributions of PRV research to herpesvirus biology, neurobiology, and viral pathogenesis by focusing on (i) the molecular biology of PRV, (ii) model systems to study PRV pathogenesis and neurovirulence, (iii) PRV transsynaptic tracing of neuronal circuits, and (iv) veterinary aspects of pseudorabies disease. The structure of the enveloped infectious particle, the content of the viral DNA genome, and a step-by-step overview of the viral replication cycle are presented. PRV infection is initiated by binding to cellular receptors to allow penetration into the cell. After reaching the nucleus, the viral genome directs a regulated gene expression cascade that culminates with viral DNA replication and production of new virion constituents. Finally, progeny virions self-assemble and exit the host cells. Animal models and neuronal culture systems developed for the study of PRV pathogenesis and neurovirulence are discussed. PRV serves asa self-perpetuating transsynaptic tracer of neuronal circuitry, and we detail the original studies of PRV circuitry mapping, the biology underlying this application, and the development of the next generation of tracer viruses. The basic veterinary aspects of pseudorabies management and disease in swine are discussed. PRV infection progresses from acute infection of the respiratory epithelium to latent infection in the peripheral nervous system. Sporadic reactivation from latency can transmit PRV to new hosts. The successful management of PRV disease has relied on vaccination, prevention, and testing.
Figures
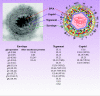
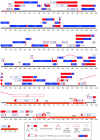
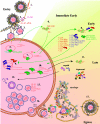
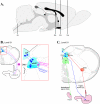
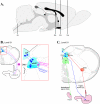
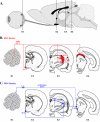
Similar articles
-
Pseudorabies virus expressing bovine herpesvirus 1 glycoprotein B exhibits altered neurotropism and increased neurovirulence.J Virol. 2000 Jan;74(2):817-27. doi: 10.1128/jvi.74.2.817-827.2000. J Virol. 2000. PMID: 10623744 Free PMC article.
-
Pseudorabies (Aujeszky's disease) virus: state of the art. August 1993.Acta Vet Hung. 1994;42(2-3):153-77. Acta Vet Hung. 1994. PMID: 7810409 Review.
-
Pseudorabies virus in wild swine: a global perspective.Arch Virol. 2011 Oct;156(10):1691-705. doi: 10.1007/s00705-011-1080-2. Epub 2011 Aug 12. Arch Virol. 2011. PMID: 21837416 Review.
-
Potential sites of virus latency associated with indigenous pseudorabies viruses in feral swine.J Wildl Dis. 2003 Jul;39(3):567-75. doi: 10.7589/0090-3558-39.3.567. J Wildl Dis. 2003. PMID: 14567217
-
Glycoprotein I of pseudorabies virus (Aujeszky's disease virus) determines virulence and facilitates penetration of the virus into the central nervous system of pigs.Acta Vet Hung. 1994;42(2-3):289-300. Acta Vet Hung. 1994. PMID: 7810422
Cited by
-
Characterization of Nucleocytoplasmic Shuttling of Pseudorabies Virus Protein UL46.Front Vet Sci. 2020 Aug 21;7:484. doi: 10.3389/fvets.2020.00484. eCollection 2020. Front Vet Sci. 2020. PMID: 32974393 Free PMC article.
-
Kaempferol: A Review of Current Evidence of Its Antiviral Potential.Int J Mol Sci. 2023 Nov 14;24(22):16299. doi: 10.3390/ijms242216299. Int J Mol Sci. 2023. PMID: 38003488 Free PMC article. Review.
-
Viral infection resistance conferred on mice by siRNA transgenesis.Transgenic Res. 2013 Jun;22(3):489-500. doi: 10.1007/s11248-012-9649-4. Epub 2012 Sep 9. Transgenic Res. 2013. PMID: 22961198
-
Host cellular factors involved in pseudorabies virus attachment and entry: a mini review.Front Vet Sci. 2023 Nov 27;10:1314624. doi: 10.3389/fvets.2023.1314624. eCollection 2023. Front Vet Sci. 2023. PMID: 38089700 Free PMC article. Review.
-
Comprehensive evaluation of the safety and immunogenicity of a gene-deleted variant pseudorabies virus attenuated vaccine.Vet Res. 2022 Sep 22;53(1):73. doi: 10.1186/s13567-022-01091-4. Vet Res. 2022. PMID: 36138470 Free PMC article.
References
-
- Abmayr, S. M., J. L. Workman, and R. G. Roeder. 1988. The pseudorabies immediate-early protein stimulates in vitro transcription by facilitating TFIID: promoter interactions. Genes Dev. 2:542-553. - PubMed
-
- Adams, R., C. Cunningham, M. D. Davison, C. A. MacLean, and A. J. Davison. 1998. Characterization of the protein encoded by gene UL49A of herpes simplex virus type 1. J. Gen. Virol. 79:813-823. - PubMed
-
- Ambagala, A. P., R. S. Gopinath, and S. Srikumaran. 2003. Inhibition of TAP by pseudorabies virus is independent of its vhs activity. Virus Res. 96:37-48. - PubMed
Publication types
MeSH terms
Grants and funding
LinkOut - more resources
Full Text Sources
Other Literature Sources