Genetic analysis of the SARS-coronavirus spike glycoprotein functional domains involved in cell-surface expression and cell-to-cell fusion
- PMID: 16099010
- PMCID: PMC7111838
- DOI: 10.1016/j.virol.2005.06.046
Genetic analysis of the SARS-coronavirus spike glycoprotein functional domains involved in cell-surface expression and cell-to-cell fusion
Abstract
The SARS-coronavirus (SARS-CoV) is the etiological agent of severe acute respiratory syndrome (SARS). The SARS-CoV spike (S) glycoprotein mediates membrane fusion events during virus entry and virus-induced cell-to-cell fusion. To delineate functional domains of the SARS-CoV S glycoprotein, single point mutations, cluster-to-lysine and cluster-to-alanine mutations, as well as carboxyl-terminal truncations were investigated in transient expression experiments. Mutagenesis of either the coiled-coil domain of the S glycoprotein amino terminal heptad repeat, the predicted fusion peptide, or an adjacent but distinct region, severely compromised S-mediated cell-to-cell fusion, while intracellular transport and cell-surface expression were not adversely affected. Surprisingly, a carboxyl-terminal truncation of 17 amino acids substantially increased S glycoprotein-mediated cell-to-cell fusion suggesting that the terminal 17 amino acids regulated the S fusogenic properties. In contrast, truncation of 26 or 39 amino acids eliminating either one or both of the two endodomain cysteine-rich motifs, respectively, inhibited cell fusion in comparison to the wild-type S. The 17 and 26 amino-acid deletions did not adversely affect S cell-surface expression, while the 39 amino-acid truncation inhibited S cell-surface expression suggesting that the membrane proximal cysteine-rich motif plays an essential role in S cell-surface expression. Mutagenesis of the acidic amino-acid cluster in the carboxyl terminus of the S glycoprotein as well as modification of a predicted phosphorylation site within the acidic cluster revealed that this amino-acid motif may play a functional role in the retention of S at cell surfaces. This genetic analysis reveals that the SARS-CoV S glycoprotein contains extracellular domains that regulate cell fusion as well as distinct endodomains that function in intracellular transport, cell-surface expression, and cell fusion.
Figures
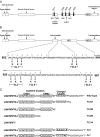
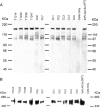
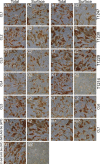
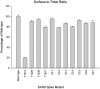
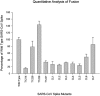
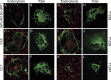
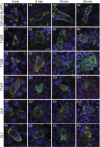

Similar articles
-
Palmitoylation of the cysteine-rich endodomain of the SARS-coronavirus spike glycoprotein is important for spike-mediated cell fusion.Virology. 2007 Apr 10;360(2):264-74. doi: 10.1016/j.virol.2006.10.034. Epub 2006 Nov 28. Virology. 2007. PMID: 17134730 Free PMC article.
-
Important role for the transmembrane domain of severe acute respiratory syndrome coronavirus spike protein during entry.J Virol. 2006 Feb;80(3):1302-10. doi: 10.1128/JVI.80.3.1302-1310.2006. J Virol. 2006. PMID: 16415007 Free PMC article.
-
Serine-scanning mutagenesis studies of the C-terminal heptad repeats in the SARS coronavirus S glycoprotein highlight the important role of the short helical region.Virology. 2005 Oct 10;341(1):122-9. doi: 10.1016/j.virol.2005.07.005. Virology. 2005. PMID: 16081124 Free PMC article.
-
The SARS-CoV S glycoprotein.Cell Mol Life Sci. 2004 Oct;61(19-20):2428-30. doi: 10.1007/s00018-004-4257-y. Cell Mol Life Sci. 2004. PMID: 15526150 Free PMC article. Review.
-
Expression, glycosylation, and modification of the spike (S) glycoprotein of SARS CoV.Methods Mol Biol. 2007;379:127-35. doi: 10.1007/978-1-59745-393-6_9. Methods Mol Biol. 2007. PMID: 17502675 Free PMC article. Review.
Cited by
-
Annotating Spike Protein Polymorphic Amino Acids of Variants of SARS-CoV-2, Including Omicron.Biochem Res Int. 2022 Apr 11;2022:2164749. doi: 10.1155/2022/2164749. eCollection 2022. Biochem Res Int. 2022. PMID: 35450296 Free PMC article.
-
Cytoplasmic Tail Truncation Stabilizes S1-S2 Association and Enhances S Protein Incorporation into SARS-CoV-2 Pseudovirions.J Virol. 2023 Mar 30;97(3):e0165022. doi: 10.1128/jvi.01650-22. Epub 2023 Feb 15. J Virol. 2023. PMID: 36790205 Free PMC article.
-
Mutation in the cytoplasmic retrieval signal of porcine epidemic diarrhea virus spike (S) protein is responsible for enhanced fusion activity.Virus Res. 2011 Nov;161(2):188-93. doi: 10.1016/j.virusres.2011.07.019. Epub 2011 Aug 5. Virus Res. 2011. PMID: 21840351 Free PMC article.
-
SARS-CoV-2 spike glycoprotein-binding proteins expressed by upper respiratory tract bacteria may prevent severe viral infection.FEBS Lett. 2020 Jun;594(11):1651-1660. doi: 10.1002/1873-3468.13845. FEBS Lett. 2020. PMID: 32449939 Free PMC article.
-
Membranotropic and biological activities of the membrane fusion peptides from SARS-CoV spike glycoprotein: The importance of the complete internal fusion peptide domain.Biochim Biophys Acta Biomembr. 2021 Nov 1;1863(11):183697. doi: 10.1016/j.bbamem.2021.183697. Epub 2021 Jul 15. Biochim Biophys Acta Biomembr. 2021. PMID: 34274319 Free PMC article.
References
-
- Aiyar A., Xiang Y., Leis J. Site-directed mutagenesis using overlap extension PCR. Methods Mol. Biol. 1996;57:177–191. - PubMed
-
- Anand K., Ziebuhr J., Wadhwani P., Mesters J.R., Hilgenfeld R. Coronavirus main proteinase (3CLpro) structure: basis for design of anti-SARS drugs. Science. 2003;300(5626):1763–1767. - PubMed
-
- Baker K.A., Dutch R.E., Lamb R.A., Jardetzky T.S. Structural basis for paramyxovirus-mediated membrane fusion. Mol. Cell. 1999;3(3):309–319. - PubMed
-
- Blom N., Gammeltoft S., Brunak S. Sequence and structure-based prediction of eukaryotic protein phosphorylation sites. J. Mol. Biol. 1999;294(5):1351–1362. - PubMed
Publication types
MeSH terms
Substances
LinkOut - more resources
Full Text Sources
Other Literature Sources
Molecular Biology Databases
Miscellaneous