Extensive syncytium formation mediated by the reovirus FAST proteins triggers apoptosis-induced membrane instability
- PMID: 15956554
- PMCID: PMC1143762
- DOI: 10.1128/JVI.79.13.8090-8100.2005
Extensive syncytium formation mediated by the reovirus FAST proteins triggers apoptosis-induced membrane instability
Abstract
The fusion-associated small transmembrane (FAST) proteins of the fusogenic reoviruses are the only known examples of membrane fusion proteins encoded by non-enveloped viruses. While the involvement of the FAST proteins in mediating extensive syncytium formation in virus-infected and -transfected cells is well established, the nature of the fusion reaction and the role of cell-cell fusion in the virus replication cycle remain unclear. To address these issues, we analyzed the syncytial phenotype induced by four different FAST proteins: the avian and Nelson Bay reovirus p10, reptilian reovirus p14, and baboon reovirus p15 FAST proteins. Results indicate that FAST protein-mediated cell-cell fusion is a relatively non-leaky process, as demonstrated by the absence of significant [3H]uridine release from cells undergoing fusion and by the resistance of these cells to treatment with hygromycin B, a membrane-impermeable translation inhibitor. However, diminished membrane integrity occurred subsequent to extensive syncytium formation and was associated with DNA fragmentation and chromatin condensation, indicating that extensive cell-cell fusion activates apoptotic signaling cascades. Inhibiting effector caspase activation or ablating the extent of syncytium formation, either by partial deletion of the avian reovirus p10 ecto-domain or by antibody inhibition of p14-mediated cell-cell fusion, all resulted in reduced membrane permeability changes. These observations suggest that the FAST proteins do not possess intrinsic membrane-lytic activity. Rather, extensive FAST protein-induced syncytium formation triggers an apoptotic response that contributes to altered membrane integrity. We propose that the FAST proteins have evolved to serve a dual role in the replication cycle of these fusogenic non-enveloped viruses, with non-leaky cell-cell fusion initially promoting localized cell-cell transmission of the infection followed by enhanced progeny virus release from apoptotic syncytia and systemic dissemination of the infection.
Figures
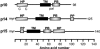
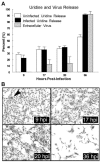
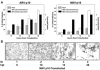
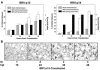
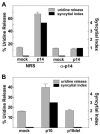
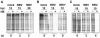
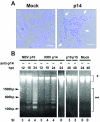
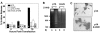
Similar articles
-
Efficient reovirus- and measles virus-mediated pore expansion during syncytium formation is dependent on annexin A1 and intracellular calcium.J Virol. 2014 Jun;88(11):6137-47. doi: 10.1128/JVI.00121-14. Epub 2014 Mar 19. J Virol. 2014. PMID: 24648446 Free PMC article.
-
Avian reovirus-induced syncytium formation is independent of infectious progeny virus production and enhances the rate, but is not essential, for virus-induced cytopathology and virus egress.Virology. 1996 Oct 15;224(2):453-64. doi: 10.1006/viro.1996.0552. Virology. 1996. PMID: 8874506
-
Multifaceted sequence-dependent and -independent roles for reovirus FAST protein cytoplasmic tails in fusion pore formation and syncytiogenesis.J Virol. 2009 Dec;83(23):12185-95. doi: 10.1128/JVI.01667-09. Epub 2009 Sep 16. J Virol. 2009. PMID: 19759162 Free PMC article.
-
Reovirus FAST proteins: virus-encoded cellular fusogens.Trends Microbiol. 2014 Dec;22(12):715-24. doi: 10.1016/j.tim.2014.08.005. Epub 2014 Sep 19. Trends Microbiol. 2014. PMID: 25245455 Review.
-
Fusogenic Reoviruses and Their Fusion-Associated Small Transmembrane (FAST) Proteins.Annu Rev Virol. 2019 Sep 29;6(1):341-363. doi: 10.1146/annurev-virology-092818-015523. Epub 2019 Jul 5. Annu Rev Virol. 2019. PMID: 31283438 Review.
Cited by
-
Cholesterol 25-hydroxylase suppresses avian reovirus replication by its enzymatic product 25-hydroxycholesterol.Front Microbiol. 2023 Jun 29;14:1178005. doi: 10.3389/fmicb.2023.1178005. eCollection 2023. Front Microbiol. 2023. PMID: 37455710 Free PMC article.
-
Cell-Cell Fusion Mediated by Viruses and HERV-Derived Fusogens in Cancer Initiation and Progression.Cancers (Basel). 2021 Oct 26;13(21):5363. doi: 10.3390/cancers13215363. Cancers (Basel). 2021. PMID: 34771528 Free PMC article. Review.
-
A new aquareovirus causing high mortality in farmed Atlantic halibut fry in Norway.Arch Virol. 2015 Jan;160(1):91-102. doi: 10.1007/s00705-014-2235-8. Epub 2014 Oct 28. Arch Virol. 2015. PMID: 25348270 Free PMC article.
-
Fusogenic vesicular stomatitis virus combined with natural killer T cell immunotherapy controls metastatic breast cancer.Breast Cancer Res. 2024 May 15;26(1):78. doi: 10.1186/s13058-024-01818-5. Breast Cancer Res. 2024. PMID: 38750591 Free PMC article.
-
Reovirus FAST Protein Enhances Vesicular Stomatitis Virus Oncolytic Virotherapy in Primary and Metastatic Tumor Models.Mol Ther Oncolytics. 2017 Aug 4;6:80-89. doi: 10.1016/j.omto.2017.08.001. eCollection 2017 Sep 15. Mol Ther Oncolytics. 2017. PMID: 28856238 Free PMC article.
References
-
- Aldabe, R., A. Barco, and L. Carrasco. 1996. Membrane permeabilization by poliovirus proteins 2B and 2BC. J. Biol. Chem. 271:23134-23137. - PubMed
-
- Bateman, A. R., K. J. Harrington, T. Kottke, A. Ahmed, A. A. Melcher, M. J. Gough, E. Linardakis, D. Riddle, A. Dietz, C. M. Lohse, S. Strome, T. Peterson, R. Simari, and R. G. Vile. 2002. Viral fusogenic membrane glycoproteins kill solid tumor cells by nonapoptotic mechanisms that promote cross presentation of tumor antigens by dendritic cells. Cancer Res. 62:6566-6578. - PubMed
-
- Black, S., M. Kadyrov, P. Kaufmann, B. Ugele, N. Emans, and B. Huppertz. 2004. Syncytial fusion of human trophoblast depends on caspase 8. Cell Death Differ. 11:90-98. - PubMed
-
- Bodelon, G., L. Labrada, J. Martinez-Costas, and J. Benavente. 2002. Modification of late membrane permeability in avian reovirus-infected cells: viroporin activity of the S1-encoded nonstructural p10 protein. J. Biol. Chem. 277:17789-17796. - PubMed
Publication types
MeSH terms
Substances
LinkOut - more resources
Full Text Sources
Other Literature Sources
Research Materials