The semi-phosphorylative Entner-Doudoroff pathway in hyperthermophilic archaea: a re-evaluation
- PMID: 15869466
- PMCID: PMC1198933
- DOI: 10.1042/BJ20041711
The semi-phosphorylative Entner-Doudoroff pathway in hyperthermophilic archaea: a re-evaluation
Abstract
Biochemical studies have suggested that, in hyperthermophilic archaea, the metabolic conversion of glucose via the ED (Entner-Doudoroff) pathway generally proceeds via a non-phosphorylative variant. A key enzyme of the non-phosphorylating ED pathway of Sulfolobus solfataricus, KDG (2-keto-3-deoxygluconate) aldolase, has been cloned and characterized previously. In the present study, a comparative genomics analysis is described that reveals conserved ED gene clusters in both Thermoproteus tenax and S. solfataricus. The corresponding ED proteins from both archaea have been expressed in Escherichia coli and their specificity has been identified, revealing: (i) a novel type of gluconate dehydratase (gad gene), (ii) a bifunctional 2-keto-3-deoxy-(6-phospho)-gluconate aldolase (kdgA gene), (iii) a 2-keto-3-deoxygluconate kinase (kdgK gene) and, in S. solfataricus, (iv) a GAPN (non-phosphorylating glyceraldehyde-3-phosphate dehydrogenase; gapN gene). Extensive in vivo and in vitro enzymatic analyses indicate the operation of both the semi-phosphorylative and the non-phosphorylative ED pathway in T. tenax and S. solfataricus. The existence of this branched ED pathway is yet another example of the versatility and flexibility of the central carbohydrate metabolic pathways in the archaeal domain.
Figures
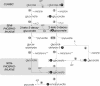
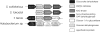
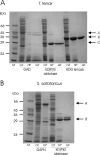
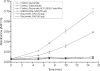
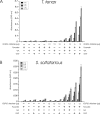
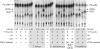
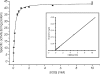
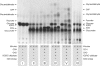
Similar articles
-
The non-phosphorylating glyceraldehyde-3-phosphate dehydrogenase (GAPN) of Sulfolobus solfataricus: a key-enzyme of the semi-phosphorylative branch of the Entner-Doudoroff pathway.Extremophiles. 2008 Jan;12(1):75-88. doi: 10.1007/s00792-007-0082-1. Epub 2007 Jun 5. Extremophiles. 2008. PMID: 17549431
-
Crystal structure and stereochemical studies of KD(P)G aldolase from Thermoproteus tenax.Proteins. 2008 Jul;72(1):35-43. doi: 10.1002/prot.21890. Proteins. 2008. PMID: 18186475
-
Promiscuity in the part-phosphorylative Entner-Doudoroff pathway of the archaeon Sulfolobus solfataricus.FEBS Lett. 2005 Dec 19;579(30):6865-9. doi: 10.1016/j.febslet.2005.11.028. Epub 2005 Dec 1. FEBS Lett. 2005. PMID: 16330030
-
The central carbohydrate metabolism of the hyperthermophilic crenarchaeote Thermoproteus tenax: pathways and insights into their regulation.Arch Microbiol. 2008 Sep;190(3):231-45. doi: 10.1007/s00203-008-0375-5. Epub 2008 May 20. Arch Microbiol. 2008. PMID: 18491075 Review.
-
Embden-Meyerhof-Parnas and Entner-Doudoroff pathways in Thermoproteus tenax: metabolic parallelism or specific adaptation?Biochem Soc Trans. 2004 Apr;32(Pt 2):303-4. doi: 10.1042/bst0320303. Biochem Soc Trans. 2004. PMID: 15046594 Review.
Cited by
-
Computation-facilitated assignment of the function in the enolase superfamily: a regiochemically distinct galactarate dehydratase from Oceanobacillus iheyensis.Biochemistry. 2009 Dec 8;48(48):11546-58. doi: 10.1021/bi901731c. Biochemistry. 2009. PMID: 19883118 Free PMC article.
-
Absence of diauxie during simultaneous utilization of glucose and Xylose by Sulfolobus acidocaldarius.J Bacteriol. 2011 Mar;193(6):1293-301. doi: 10.1128/JB.01219-10. Epub 2011 Jan 14. J Bacteriol. 2011. PMID: 21239580 Free PMC article.
-
The non-phosphorylating glyceraldehyde-3-phosphate dehydrogenase (GAPN) of Sulfolobus solfataricus: a key-enzyme of the semi-phosphorylative branch of the Entner-Doudoroff pathway.Extremophiles. 2008 Jan;12(1):75-88. doi: 10.1007/s00792-007-0082-1. Epub 2007 Jun 5. Extremophiles. 2008. PMID: 17549431
-
d-Ribose Catabolism in Archaea: Discovery of a Novel Oxidative Pathway in Haloarcula Species.J Bacteriol. 2020 Jan 15;202(3):e00608-19. doi: 10.1128/JB.00608-19. Print 2020 Jan 15. J Bacteriol. 2020. PMID: 31712277 Free PMC article.
-
Cloning, gene expression and characterization of a novel bacterial NAD-dependent non-phosphorylating glyceraldehyde-3-phosphate dehydrogenase from Neisseria meningitidis strain Z2491.Mol Cell Biochem. 2007 Nov;305(1-2):209-19. doi: 10.1007/s11010-007-9545-z. Epub 2007 Jul 10. Mol Cell Biochem. 2007. PMID: 17619949
References
Publication types
MeSH terms
Substances
LinkOut - more resources
Full Text Sources
Molecular Biology Databases
Research Materials