Dictyostelium LIS1 is a centrosomal protein required for microtubule/cell cortex interactions, nucleus/centrosome linkage, and actin dynamics
- PMID: 15800059
- PMCID: PMC1142422
- DOI: 10.1091/mbc.e05-01-0069
Dictyostelium LIS1 is a centrosomal protein required for microtubule/cell cortex interactions, nucleus/centrosome linkage, and actin dynamics
Abstract
The widespread LIS1-proteins were originally identified as the target for sporadic mutations causing lissencephaly in humans. Dictyostelium LIS1 (DdLIS1) is a microtubule-associated protein exhibiting 53% identity to human LIS1. It colocalizes with dynein at isolated, microtubule-free centrosomes, suggesting that both are integral centrosomal components. Replacement of the DdLIS1 gene by the hypomorphic D327H allele or overexpression of an MBP-DdLIS1 fusion disrupted various dynein-associated functions. Microtubules lost contact with the cell cortex and were dragged behind an unusually motile centrosome. Previously, this phenotype was observed in cells overexpressing fragments of dynein or the XMAP215-homologue DdCP224. DdLIS1 was coprecipitated with DdCP224, suggesting that both act together in dynein-mediated cortical attachment of microtubules. Furthermore, DdLIS1-D327H mutants showed Golgi dispersal and reduced centrosome/nucleus association. Defects in DdLIS1 function also altered actin dynamics characterized by traveling waves of actin polymerization correlated with a reduced F-actin content. DdLIS1 could be involved in actin dynamics through Rho-GTPases, because DdLIS1 interacted directly with Rac1A in vitro. Our results show that DdLIS1 is required for maintenance of the microtubule cytoskeleton, Golgi apparatus and nucleus/centrosome association, and they suggest that LIS1-dependent alterations of actin dynamics could also contribute to defects in neuronal migration in lissencephaly patients.
Figures
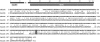
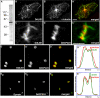
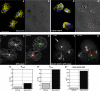
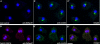
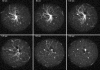
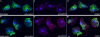
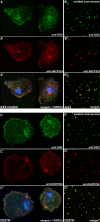
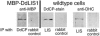
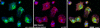
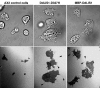
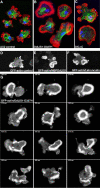
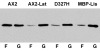
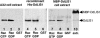
Similar articles
-
The XMAP215-family protein DdCP224 is required for cortical interactions of microtubules.BMC Cell Biol. 2004 Jun 8;5:24. doi: 10.1186/1471-2121-5-24. BMC Cell Biol. 2004. PMID: 15186508 Free PMC article.
-
Centrosomal microtubule plus end tracking proteins and their role in Dictyostelium cell dynamics.J Muscle Res Cell Motil. 2002;23(7-8):621-30. doi: 10.1023/a:1024450922609. J Muscle Res Cell Motil. 2002. PMID: 12952061 Review.
-
Dictyostelium DdCP224 is a microtubule-associated protein and a permanent centrosomal resident involved in centrosome duplication.J Cell Sci. 2000 May;113 ( Pt 10):1747-58. doi: 10.1242/jcs.113.10.1747. J Cell Sci. 2000. PMID: 10769206
-
Regulated expression of the centrosomal protein DdCP224 affects microtubule dynamics and reveals mechanisms for the control of supernumerary centrosome number.Mol Biol Cell. 2003 Oct;14(10):4067-74. doi: 10.1091/mbc.e03-04-0242. Epub 2003 Jun 13. Mol Biol Cell. 2003. PMID: 14517319 Free PMC article.
-
Functional analyses of lissencephaly-related proteins in Dictyostelium.Semin Cell Dev Biol. 2011 Feb;22(1):89-96. doi: 10.1016/j.semcdb.2010.10.007. Epub 2010 Oct 27. Semin Cell Dev Biol. 2011. PMID: 21034843 Review.
Cited by
-
A kinesin-mediated mechanism that couples centrosomes to nuclei.Cell Mol Life Sci. 2013 Apr;70(7):1285-96. doi: 10.1007/s00018-012-1205-0. Epub 2012 Nov 17. Cell Mol Life Sci. 2013. PMID: 23161062 Free PMC article.
-
A lamin in lower eukaryotes?Nucleus. 2012 May-Jun;3(3):237-43. doi: 10.4161/nucl.20149. Epub 2012 May 1. Nucleus. 2012. PMID: 22572958 Free PMC article. Review.
-
The Golgi and the centrosome: building a functional partnership.J Cell Biol. 2010 Mar 8;188(5):621-8. doi: 10.1083/jcb.200910001. J Cell Biol. 2010. PMID: 20212314 Free PMC article. Review.
-
Activation of RhoC by regulatory ubiquitination is mediated by LNX1 and suppressed by LIS1.Sci Rep. 2022 Oct 3;12(1):16493. doi: 10.1038/s41598-022-19740-1. Sci Rep. 2022. PMID: 36192543 Free PMC article.
-
Functional characterization of CP148, a novel key component for centrosome integrity in Dictyostelium.Cell Mol Life Sci. 2012 Jun;69(11):1875-88. doi: 10.1007/s00018-011-0904-2. Epub 2012 Jan 6. Cell Mol Life Sci. 2012. PMID: 22223109 Free PMC article.
References
-
- Bretschneider, T., Diez, S., Anderson, K., Heuser, J., Clarke, M., Müller-Taubenberger, A., Köhler, J., and Gerisch, G. (2004). Dynamic actin patterns and Arp2/3 assembly at the substrate-attached surface of motile cells. Curr. Biol. 14, 1–10. - PubMed
-
- Bush, J., Franek, K., and Cardelli, J. (1993). Cloning and characterization of seven novel Dictyostelium discoideum rac-related genes belonging to the rho family of GTPases. Gene 136, 61–68. - PubMed
-
- Caspi, M., Atlas, R., Kantor, A., Sapir, T., and Reiner, O. (2000). Interaction between LIS1 and doublecortin, two lissencephaly gene products. Hum. Mol. Genet. 9, 2205–2213. - PubMed
-
- Caspi, M., Coquelle, F. M., Koifman, C., Levy, T., Arai, H., Aoki, J., De Mey, J. R., and Reiner, O. (2003). LIS1 missense mutations: variable phenotypes result from unpredictable alterations in biochemical and cellular properties. J. Biol. Chem. 278, 38740–38748. - PubMed
Publication types
MeSH terms
Substances
LinkOut - more resources
Full Text Sources
Molecular Biology Databases
Research Materials
Miscellaneous