Effects of {gamma}-tubulin complex proteins on microtubule nucleation and catastrophe in fission yeast
- PMID: 15772152
- PMCID: PMC1142419
- DOI: 10.1091/mbc.e04-08-0676
Effects of {gamma}-tubulin complex proteins on microtubule nucleation and catastrophe in fission yeast
Abstract
Although gamma-tubulin complexes (gamma-TuCs) are known as microtubule (MT) nucleators, their function in vivo is still poorly defined. Mto1p (also known as mbo1p or mod20p) is a gamma-TuC-associated protein that recruits gamma-TuCs specifically to cytoplasmic MT organizing centers (MTOCs) and interphase MTs. Here, we investigated gamma-TuC function by analyzing MT behavior in mto1Delta and alp4 (GCP2 homologue) mutants. These cells have free, extra-long interphase MTs that exhibit abnormal behaviors such as cycles of growth and breakage, MT sliding, treadmilling, and hyperstability. The plus ends of interphase and spindle MTs grow continuously, exhibiting catastrophe defects that are dependent on the CLIP170 tip1p. The minus ends of interphase MTs exhibit shrinkage and pauses. As mto1Delta mutants lack cytoplasmic MTOCs, cytoplasmic MTs arise from spindle or other intranuclear MTs that exit the nucleus. Our findings show that mto1p and gamma-TuCs affect multiple properties of MTs including nucleation, nuclear attachment, plus-end catastrophe, and minus-end shrinkage.
Figures
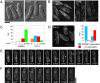
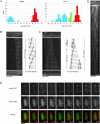
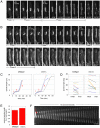
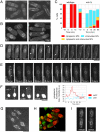
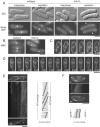
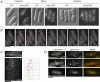
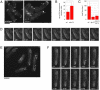
Similar articles
-
Fission yeast MOZART1/Mzt1 is an essential γ-tubulin complex component required for complex recruitment to the microtubule organizing center, but not its assembly.Mol Biol Cell. 2013 Sep;24(18):2894-906. doi: 10.1091/mbc.E13-05-0235. Epub 2013 Jul 24. Mol Biol Cell. 2013. PMID: 23885124 Free PMC article.
-
Fission yeast mto2p regulates microtubule nucleation by the centrosomin-related protein mto1p.Mol Biol Cell. 2005 Jun;16(6):3040-51. doi: 10.1091/mbc.e04-11-1003. Epub 2005 Jan 19. Mol Biol Cell. 2005. PMID: 15659644 Free PMC article.
-
Microtubule stabilization in vivo by nucleation-incompetent γ-tubulin complex.J Cell Sci. 2011 Apr 15;124(Pt 8):1207-13. doi: 10.1242/jcs.083741. J Cell Sci. 2011. PMID: 21444751 Free PMC article.
-
Targeting of γ-tubulin complexes to microtubule organizing centers: conservation and divergence.Trends Cell Biol. 2015 May;25(5):296-307. doi: 10.1016/j.tcb.2014.12.002. Epub 2014 Dec 24. Trends Cell Biol. 2015. PMID: 25544667 Review.
-
Microtubule nucleation for the assembly of acentrosomal microtubule arrays in plant cells.New Phytol. 2019 Jun;222(4):1705-1718. doi: 10.1111/nph.15705. Epub 2019 Feb 25. New Phytol. 2019. PMID: 30681146 Review.
Cited by
-
A stable microtubule array drives fission yeast polarity reestablishment upon quiescence exit.J Cell Biol. 2015 Jul 6;210(1):99-113. doi: 10.1083/jcb.201502025. Epub 2015 Jun 29. J Cell Biol. 2015. PMID: 26124291 Free PMC article.
-
CDK5RAP2 regulates centriole engagement and cohesion in mice.Dev Cell. 2010 Jun 15;18(6):913-26. doi: 10.1016/j.devcel.2010.05.017. Dev Cell. 2010. PMID: 20627074 Free PMC article.
-
Timely septation requires SNAD-dependent spindle pole body localization of the septation initiation network components in the filamentous fungus Aspergillus nidulans.Mol Biol Cell. 2009 Jun;20(12):2874-84. doi: 10.1091/mbc.e08-12-1177. Epub 2009 Apr 22. Mol Biol Cell. 2009. PMID: 19386763 Free PMC article.
-
The XMAP215 Ortholog Alp14 Promotes Microtubule Nucleation in Fission Yeast.Curr Biol. 2018 Jun 4;28(11):1681-1691.e4. doi: 10.1016/j.cub.2018.04.008. Epub 2018 May 17. Curr Biol. 2018. PMID: 29779879 Free PMC article.
-
Latrunculin A delays anaphase onset in fission yeast by disrupting an Ase1-independent pathway controlling mitotic spindle stability.Mol Biol Cell. 2008 Sep;19(9):3713-23. doi: 10.1091/mbc.e08-02-0164. Epub 2008 Jun 18. Mol Biol Cell. 2008. PMID: 18562692 Free PMC article.
References
-
- Bahler, J., Wu, J. Q., Longtine, M. S., Shah, N. G., McKenzie, A., 3rd, Steever, A. B., Wach, A., Philippsen, P., and Pringle, J. R. (1998). Heterologous modules for efficient and versatile PCR-based gene targeting in Schizosaccharomyces pombe. Yeast 14, 943–951. - PubMed
-
- Brunner, D., and Nurse, P. (2000). CLIP170-like tip1p spatially organizes microtubular dynamics in fission yeast. Cell 102, 695–704. - PubMed
-
- Chretien, D., and Fuller, S. D. (2000). Microtubules switch occasionally into unfavorable configurations during elongation. J. Mol. Biol. 298, 663–676. - PubMed
Publication types
MeSH terms
Substances
Grants and funding
LinkOut - more resources
Full Text Sources
Molecular Biology Databases
Research Materials