Binding of microtubule-associated protein 1B to LIS1 affects the interaction between dynein and LIS1
- PMID: 15762842
- PMCID: PMC1175110
- DOI: 10.1042/BJ20050244
Binding of microtubule-associated protein 1B to LIS1 affects the interaction between dynein and LIS1
Abstract
For neuronal migration to occur, the cell must undergo morphological changes that require modifications of the cytoskeleton. Several different MAPs (microtubule-associated proteins) or actin-binding proteins are proposed to be involved in the migration of neurons. Therefore we have specifically analysed how two members of the MAP family, MAP1B and LIS1 (lissencephaly-related protein 1), interact with one another and participate in neuronal migration. Our results indicate that, in hippocampal neurons, MAP1B and LIS1 co-localize, associate and interact with each another. The interaction between these two MAPs is regulated by the phosphorylation of MAP1B. Furthermore, this interaction interferes with the association between LIS1 and the microtubule-dependent molecular motor, dynein. Clearly, the differential binding of these cytoskeletal proteins could regulate the functions attributed to the LIS1-dynein complex, including those related to extension of the neural processes necessary for neuronal migration.
Figures
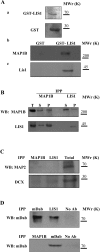
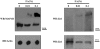
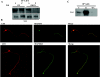
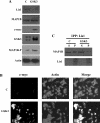
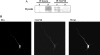
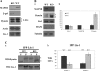
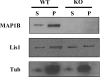
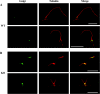
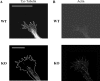
Similar articles
-
Opposing effects of Ndel1 and alpha1 or alpha2 on cytoplasmic dynein through competitive binding to Lis1.J Cell Sci. 2009 Aug 15;122(Pt 16):2820-7. doi: 10.1242/jcs.048777. Epub 2009 Jul 21. J Cell Sci. 2009. PMID: 19622634
-
[Molecular mechanism of lissencephaly--how LIS1 and NDEL1 regulate cytoplasmic dynein?].Brain Nerve. 2008 Apr;60(4):375-81. Brain Nerve. 2008. PMID: 18421979 Review. Japanese.
-
LIS1 functions in normal development and disease.Curr Opin Neurobiol. 2013 Dec;23(6):951-6. doi: 10.1016/j.conb.2013.08.001. Epub 2013 Aug 23. Curr Opin Neurobiol. 2013. PMID: 23973156 Review.
-
Ndel1 operates in a common pathway with LIS1 and cytoplasmic dynein to regulate cortical neuronal positioning.Neuron. 2004 Oct 14;44(2):263-77. doi: 10.1016/j.neuron.2004.09.030. Neuron. 2004. PMID: 15473966
-
Lis1 and doublecortin function with dynein to mediate coupling of the nucleus to the centrosome in neuronal migration.J Cell Biol. 2004 Jun 7;165(5):709-21. doi: 10.1083/jcb.200309025. Epub 2004 Jun 1. J Cell Biol. 2004. PMID: 15173193 Free PMC article.
Cited by
-
Lissencephaly 1 linking to multiple diseases: mental retardation, neurodegeneration, schizophrenia, male sterility, and more.Neuromolecular Med. 2006;8(4):547-65. doi: 10.1385/NMM:8:4:547. Neuromolecular Med. 2006. PMID: 17028375 Review.
-
The p150(Glued) CAP-Gly domain regulates initiation of retrograde transport at synaptic termini.Neuron. 2012 Apr 26;74(2):344-60. doi: 10.1016/j.neuron.2012.02.026. Neuron. 2012. PMID: 22542187 Free PMC article.
-
Cytoskeleton in action: lissencephaly, a neuronal migration disorder.Wiley Interdiscip Rev Dev Biol. 2013 Mar-Apr;2(2):229-45. doi: 10.1002/wdev.67. Wiley Interdiscip Rev Dev Biol. 2013. PMID: 23495356 Free PMC article. Review.
-
LIS1 and DCX: Implications for Brain Development and Human Disease in Relation to Microtubules.Scientifica (Cairo). 2013;2013:393975. doi: 10.1155/2013/393975. Epub 2013 Mar 17. Scientifica (Cairo). 2013. PMID: 24278775 Free PMC article. Review.
-
WD40-Repeat Proteins in Ciliopathies and Congenital Disorders of Endocrine System.Endocrinol Metab (Seoul). 2020 Sep;35(3):494-506. doi: 10.3803/EnM.2020.302. Epub 2020 Sep 8. Endocrinol Metab (Seoul). 2020. PMID: 32894826 Free PMC article. Review.
References
-
- Tucker R. P., Garner C. C., Matus A. In situ localization of microtubule-associated protein mRNA in the developing and adult rat brain. Neuron. 1989;2:1245–1256. - PubMed
-
- Diaz-Nido J., Avila J. Characterization of proteins immunologically related to brain microtubule-associated protein MAP-1B in non-neural cells. J. Cell Sci. 1989;92:607–620. - PubMed
-
- Roos J., Hummel T., Ng N., Klambt C., Davis G. W. Drosophila Futsch regulates synaptic microtubule organization and is necessary for synaptic growth. Neuron. 2000;26:371–382. - PubMed
-
- Gonzalez-Billault C., Demandt E., Wandosell F., Torres M., Bonaldo P., Stoykova A., Chowdhury K., Gruss P., Avila J., Sanchez M. P. Perinatal lethality of microtubule-associated protein 1B-deficient mice expressing alternative isoforms of the protein at low levels. Mol. Cell. Neurosci. 2000;16:408–421. - PubMed
Publication types
MeSH terms
Substances
LinkOut - more resources
Full Text Sources
Research Materials
Miscellaneous