Initiation of protein synthesis in bacteria
- PMID: 15755955
- PMCID: PMC1082788
- DOI: 10.1128/MMBR.69.1.101-123.2005
Initiation of protein synthesis in bacteria
Abstract
Valuable information on translation initiation is available from biochemical data and recently solved structures. We present a detailed description of current knowledge about the structure, function, and interactions of the individual components involved in bacterial translation initiation. The first section describes the ribosomal features relevant to the initiation process. Subsequent sections describe the structure, function, and interactions of the mRNA, the initiator tRNA, and the initiation factors IF1, IF2, and IF3. Finally, we provide an overview of mechanisms of regulation of the translation initiation event. Translation occurs on ribonucleoprotein complexes called ribosomes. The ribosome is composed of a large subunit and a small subunit that hold the activities of peptidyltransfer and decode the triplet code of the mRNA, respectively. Translation initiation is promoted by IF1, IF2, and IF3, which mediate base pairing of the initiator tRNA anticodon to the mRNA initiation codon located in the ribosomal P-site. The mechanism of translation initiation differs for canonical and leaderless mRNAs, since the latter is dependent on the relative level of the initiation factors. Regulation of translation occurs primarily in the initiation phase. Secondary structures at the mRNA ribosomal binding site (RBS) inhibit translation initiation. The accessibility of the RBS is regulated by temperature and binding of small metabolites, proteins, or antisense RNAs. The future challenge is to obtain atomic-resolution structures of complete initiation complexes in order to understand the mechanism of translation initiation in molecular detail.
Figures
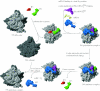
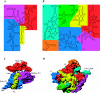
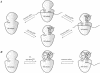
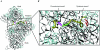
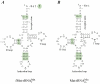
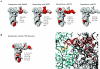
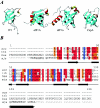
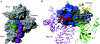
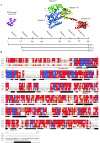
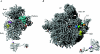
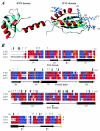
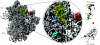
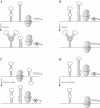
Similar articles
-
Isolation and characterization of ribosomes and translation initiation factors from the gram-positive soil bacterium Streptomyces lividans.J Bacteriol. 2004 Oct;186(20):6864-75. doi: 10.1128/JB.186.20.6864-6875.2004. J Bacteriol. 2004. PMID: 15466040 Free PMC article.
-
A leaderless mRNA can bind to mammalian 80S ribosomes and direct polypeptide synthesis in the absence of translation initiation factors.Mol Cell Biol. 2006 Apr;26(8):3164-9. doi: 10.1128/MCB.26.8.3164-3169.2006. Mol Cell Biol. 2006. PMID: 16581790 Free PMC article.
-
Modulation of ribosomal recruitment to 5'-terminal start codons by translation initiation factors IF2 and IF3.FEBS Lett. 2001 Apr 27;495(3):167-71. doi: 10.1016/s0014-5793(01)02378-x. FEBS Lett. 2001. PMID: 11334885
-
A structural view of translation initiation in bacteria.Cell Mol Life Sci. 2009 Feb;66(3):423-36. doi: 10.1007/s00018-008-8416-4. Cell Mol Life Sci. 2009. PMID: 19011758 Free PMC article. Review.
-
Initiation of mRNA translation in bacteria: structural and dynamic aspects.Cell Mol Life Sci. 2015 Nov;72(22):4341-67. doi: 10.1007/s00018-015-2010-3. Epub 2015 Aug 11. Cell Mol Life Sci. 2015. PMID: 26259514 Free PMC article. Review.
Cited by
-
In vivo biochemistry in bacterial cells using FRAP: insight into the translation cycle.Biophys J. 2012 Nov 7;103(9):1848-59. doi: 10.1016/j.bpj.2012.09.035. Biophys J. 2012. PMID: 23199913 Free PMC article.
-
Ribosomal initiation complex-driven changes in the stability and dynamics of initiation factor 2 regulate the fidelity of translation initiation.J Mol Biol. 2015 May 8;427(9):1819-34. doi: 10.1016/j.jmb.2014.12.025. Epub 2015 Jan 14. J Mol Biol. 2015. PMID: 25596426 Free PMC article.
-
Explorations of catalytic domains in non-ribosomal peptide synthetase enzymology.Nat Prod Rep. 2012 Oct;29(10):1074-98. doi: 10.1039/c2np20025b. Epub 2012 Jul 17. Nat Prod Rep. 2012. PMID: 22802156 Free PMC article. Review.
-
Position of eukaryotic initiation factor eIF5B on the 80S ribosome mapped by directed hydroxyl radical probing.EMBO J. 2007 Jul 11;26(13):3109-23. doi: 10.1038/sj.emboj.7601751. Epub 2007 Jun 14. EMBO J. 2007. PMID: 17568775 Free PMC article.
-
The effect of phenobarbital treatment on behavioral comorbidities and on the composition and function of the fecal microbiome in dogs with idiopathic epilepsy.Front Vet Sci. 2022 Aug 4;9:933905. doi: 10.3389/fvets.2022.933905. eCollection 2022. Front Vet Sci. 2022. PMID: 35990279 Free PMC article.
References
-
- Adhin, M. R., and J. van Duin. 1990. Scanning model for translational reinitiation in eubacteria. J. Mol. Biol. 213:811-818. - PubMed
-
- Ban, N., P. Nissen, J. Hansen, P. B. Moore, and T. A. Steitz. 2000. The complete atomic structure of the large ribosomal subunit at 2.4 A resolution. Science 289:905-920. - PubMed
Publication types
MeSH terms
LinkOut - more resources
Full Text Sources
Other Literature Sources
Molecular Biology Databases