Clonally expanded novel multipotent stem cells from human bone marrow regenerate myocardium after myocardial infarction
- PMID: 15690083
- PMCID: PMC546424
- DOI: 10.1172/JCI22326
Clonally expanded novel multipotent stem cells from human bone marrow regenerate myocardium after myocardial infarction
Abstract
We have identified a subpopulation of stem cells within adult human BM, isolated at the single-cell level, that self-renew without loss of multipotency for more than 140 population doublings and exhibit the capacity for differentiation into cells of all 3 germ layers. Based on surface marker expression, these clonally expanded human BM-derived multipotent stem cells (hBMSCs) do not appear to belong to any previously described BM-derived stem cell population. Intramyocardial transplantation of hBMSCs after myocardial infarction resulted in robust engraftment of transplanted cells, which exhibited colocalization with markers of cardiomyocyte (CMC), EC, and smooth muscle cell (SMC) identity, consistent with differentiation of hBMSCs into multiple lineages in vivo. Furthermore, upregulation of paracrine factors including angiogenic cytokines and antiapoptotic factors, and proliferation of host ECs and CMCs, were observed in the hBMSC-transplanted hearts. Coculture of hBMSCs with CMCs, ECs, or SMCs revealed that phenotypic changes of hBMSCs result from both differentiation and fusion. Collectively, the favorable effect of hBMSC transplantation after myocardial infarction appears to be due to augmentation of proliferation and preservation of host myocardial tissues as well as differentiation of hBMSCs for tissue regeneration and repair. To our knowledge, this is the first demonstration that a specific population of multipotent human BM-derived stem cells can induce both therapeutic neovascularization and endogenous and exogenous cardiomyogenesis.
Figures
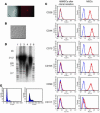
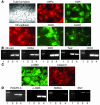
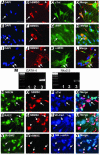
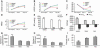
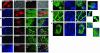
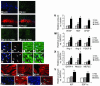
Similar articles
-
Purified human bone marrow multipotent mesenchymal stem cells regenerate infarcted myocardium in experimental rats.Cell Transplant. 2005;14(10):787-98. doi: 10.3727/000000005783982558. Cell Transplant. 2005. PMID: 16454353
-
Direct delivery of syngeneic and allogeneic large-scale expanded multipotent adult progenitor cells improves cardiac function after myocardial infarct.Cytotherapy. 2007;9(5):477-87. doi: 10.1080/14653240701452065. Cytotherapy. 2007. PMID: 17786609
-
Stem cells--clinical application and perspectives.Herz. 2002 Nov;27(7):611-20. doi: 10.1007/s00059-002-2435-y. Herz. 2002. PMID: 12439633
-
Myocardial regeneration with bone-marrow-derived stem cells.Biol Cell. 2005 Apr;97(4):253-63. doi: 10.1042/BC20040099. Biol Cell. 2005. PMID: 15762847 Review.
-
Cardiac regeneration.J Am Coll Cardiol. 2006 May 2;47(9):1769-76. doi: 10.1016/j.jacc.2006.02.003. Epub 2006 Apr 17. J Am Coll Cardiol. 2006. PMID: 16682300 Review.
Cited by
-
Cord lining-mesenchymal stem cells graft supplemented with an omental flap induces myocardial revascularization and ameliorates cardiac dysfunction in a rat model of chronic ischemic heart failure.Tissue Eng Part A. 2013 Jun;19(11-12):1303-15. doi: 10.1089/ten.tea.2012.0407. Epub 2013 Feb 28. Tissue Eng Part A. 2013. PMID: 23448654 Free PMC article.
-
Mesenchymal stem cell mediates cardiac repair through autocrine, paracrine and endocrine axes.J Transl Med. 2020 Sep 1;18(1):336. doi: 10.1186/s12967-020-02504-8. J Transl Med. 2020. PMID: 32873307 Free PMC article. Review.
-
Identification of a bone marrow-derived mesenchymal progenitor cell subset that can contribute to the gastric epithelium.Lab Invest. 2009 Dec;89(12):1410-22. doi: 10.1038/labinvest.2009.88. Epub 2009 Oct 19. Lab Invest. 2009. PMID: 19841619 Free PMC article.
-
Cell-based approaches for cardiac repair.Ann N Y Acad Sci. 2006 Oct;1080:34-48. doi: 10.1196/annals.1380.004. Ann N Y Acad Sci. 2006. PMID: 17132773 Free PMC article. Review.
-
Differentiation of rat multipotent adult progenitor cells to functional hepatocyte-like cells by mimicking embryonic liver development.Nat Protoc. 2010 Jul;5(7):1324-36. doi: 10.1038/nprot.2010.80. Epub 2010 Jun 24. Nat Protoc. 2010. PMID: 20595960
References
-
- Braunwald E, Bristow MR. Congestive heart failure: fifty years of progress. Circulation. 2000;102:IV14–IV23. - PubMed
-
- Pfeffer MA. Left ventricular remodeling after acute myocardial infarction. Annu. Rev. Med. 1995;46:455–466. - PubMed
-
- Brazelton TR, Rossi FM, Keshet GI, Blau HM. From marrow to brain: expression of neuronal phenotypes in adult mice. Science. 2000;290:1775–1779. - PubMed
-
- Mezey E, Chandross KJ, Harta G, Maki RA, McKercher SR. Turning blood into brain: cells bearing neuronal antigens generated in vivo from bone marrow. Science. 2000;290:1779–1782. - PubMed
-
- Ferrari G, et al. Muscle regeneration by bone marrow-derived myogenic progenitors. Science. 1998;279:1528–1530. - PubMed
Publication types
MeSH terms
Grants and funding
LinkOut - more resources
Full Text Sources
Other Literature Sources
Medical