Pathogenesis of persistent lymphatic vessel hyperplasia in chronic airway inflammation
- PMID: 15668734
- PMCID: PMC544601
- DOI: 10.1172/JCI22037
Pathogenesis of persistent lymphatic vessel hyperplasia in chronic airway inflammation
Abstract
Edema occurs in asthma and other inflammatory diseases when the rate of plasma leakage from blood vessels exceeds the drainage through lymphatic vessels and other routes. It is unclear to what extent lymphatic vessels grow to compensate for increased leakage during inflammation and what drives the lymphangiogenesis that does occur. We addressed these issues in mouse models of (a) chronic respiratory tract infection with Mycoplasma pulmonis and (b) adenoviral transduction of airway epithelium with VEGF family growth factors. Blood vessel remodeling and lymphangiogenesis were both robust in infected airways. Inhibition of VEGFR-3 signaling completely prevented the growth of lymphatic vessels but not blood vessels. Lack of lymphatic growth exaggerated mucosal edema and reduced the hypertrophy of draining lymph nodes. Airway dendritic cells, macrophages, neutrophils, and epithelial cells expressed the VEGFR-3 ligands VEGF-C or VEGF-D. Adenoviral delivery of either VEGF-C or VEGF-D evoked lymphangiogenesis without angiogenesis, whereas adenoviral VEGF had the opposite effect. After antibiotic treatment of the infection, inflammation and remodeling of blood vessels quickly subsided, but lymphatic vessels persisted. Together, these findings suggest that when lymphangiogenesis is impaired, airway inflammation may lead to bronchial lymphedema and exaggerated airflow obstruction. Correction of defective lymphangiogenesis may benefit the treatment of asthma and other inflammatory airway diseases.
Figures
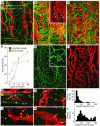
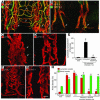
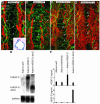
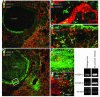
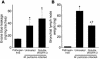
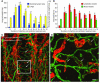
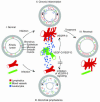
Similar articles
-
Transgenic induction of vascular endothelial growth factor-C is strongly angiogenic in mouse embryos but leads to persistent lymphatic hyperplasia in adult tissues.Am J Pathol. 2008 Dec;173(6):1891-901. doi: 10.2353/ajpath.2008.080378. Epub 2008 Nov 6. Am J Pathol. 2008. PMID: 18988807 Free PMC article.
-
Differential Receptor Binding and Regulatory Mechanisms for the Lymphangiogenic Growth Factors Vascular Endothelial Growth Factor (VEGF)-C and -D.J Biol Chem. 2016 Dec 30;291(53):27265-27278. doi: 10.1074/jbc.M116.736801. Epub 2016 Nov 16. J Biol Chem. 2016. PMID: 27852824 Free PMC article.
-
Differential expression of VEGF ligands and receptors in prostate cancer.Prostate. 2013 May;73(6):563-72. doi: 10.1002/pros.22596. Epub 2012 Oct 4. Prostate. 2013. PMID: 23038639
-
Molecular control of lymphatic metastasis.Ann N Y Acad Sci. 2008;1131:225-34. doi: 10.1196/annals.1413.020. Ann N Y Acad Sci. 2008. PMID: 18519975 Review.
-
Roles of the TGF-β⁻VEGF-C Pathway in Fibrosis-Related Lymphangiogenesis.Int J Mol Sci. 2018 Aug 23;19(9):2487. doi: 10.3390/ijms19092487. Int J Mol Sci. 2018. PMID: 30142879 Free PMC article. Review.
Cited by
-
Lymphatic system: an active pathway for immune protection.Semin Cell Dev Biol. 2015 Feb;38:83-9. doi: 10.1016/j.semcdb.2014.11.012. Epub 2014 Dec 19. Semin Cell Dev Biol. 2015. PMID: 25534659 Free PMC article. Review.
-
The Molecular Signature Related to Local Inflammatory and Immune Response in Canine Cutaneous Hypersensitivity Reactions: A Preliminary Study.Curr Issues Mol Biol. 2024 Aug 22;46(8):9162-9178. doi: 10.3390/cimb46080542. Curr Issues Mol Biol. 2024. PMID: 39194759 Free PMC article.
-
Bimodal Expansion of the Lymphatic Vessels Is Regulated by the Sequential Expression of IL-7 and Lymphotoxin α1β2 in Newly Formed Tertiary Lymphoid Structures.J Immunol. 2016 Sep 1;197(5):1957-67. doi: 10.4049/jimmunol.1500686. Epub 2016 Jul 29. J Immunol. 2016. PMID: 27474071 Free PMC article.
-
Differential changes in end organ immune cells and inflammation in salt-sensitive hypertension: effects of increasing M2 macrophages.Clin Sci (Lond). 2024 Jul 17;138(14):921-940. doi: 10.1042/CS20240699. Clin Sci (Lond). 2024. PMID: 38949840 Free PMC article.
-
Lymphangiogenesis in rat asthma model.Angiogenesis. 2017 Feb;20(1):73-84. doi: 10.1007/s10456-016-9529-2. Epub 2016 Oct 27. Angiogenesis. 2017. PMID: 27787629 Free PMC article.
References
-
- Wiggs BR, Bosken C, Pare PD, James A, Hogg JC. A model of airway narrowing in asthma and in chronic obstructive pulmonary disease. Am. Rev. Respir. Dis. 1992;145:1251–1258. - PubMed
-
- Wilson J. The bronchial microcirculation in asthma. Clin. Exp. Allergy. 2000;30:51–53. - PubMed
-
- Pullinger BD, Florey HW. Proliferation of lymphatics in inflammation. J. Pathol. Bact. 1937;45:157–170.
-
- Jussila L, Alitalo K. Vascular growth factors and lymphangiogenesis. Physiol. Rev. 2002;82:673–700. - PubMed
-
- Saaristo A, et al. Adenoviral VEGF-C overexpression induces blood vessel enlargement, tortuosity, and leakiness but no sprouting angiogenesis in the skin or mucous membranes. FASEB J. 2002;16:1041–1049. - PubMed
Publication types
MeSH terms
Substances
Grants and funding
LinkOut - more resources
Full Text Sources
Other Literature Sources
Miscellaneous