High affinity RNA for mammalian initiation factor 4E interferes with mRNA-cap binding and inhibits translation
- PMID: 15611299
- PMCID: PMC1370693
- DOI: 10.1261/rna.7108205
High affinity RNA for mammalian initiation factor 4E interferes with mRNA-cap binding and inhibits translation
Abstract
The eukaryotic translation initiation factor 4F (eIF4F) consists of three polypeptides (eIF4A, eIF4G, and eIF4E) and is responsible for recruiting ribosomes to mRNA. eIF4E recognizes the mRNA 5'-cap structure (m7GpppN) and plays a pivotal role in control of translation initiation, which is the rate-limiting step in translation. Overexpression of eIF4E has a dramatic effect on cell growth and leads to oncogenic transformation. Therefore, an inhibitory agent to eIF4E, if any, might serve as a novel therapeutic against malignancies that are caused by aberrant translational control. Along these lines, we developed two RNA aptamers, aptamer 1 and aptamer 2, with high affinity for mammalian eIF4E by in vitro RNA selection-amplification. Aptamer 1 inhibits the cap binding to eIF4E more efficiently than the cap analog m7GpppN or aptamer 2. Consistently, aptamer 1 inhibits specifically cap-dependent in vitro translation while it does not inhibit cap-independent HCV IRES-directed translation initiation. The interaction between eIF4E and eIF4E-binding protein 1 (4E-BP1), however, was not inhibited by aptamer 1. Aptamer 1 is composed of 86 nucleotides, and the high affinity to eIF4E is affected by deletions at both termini. Moreover, relatively large areas in the aptamer 1 fold are protected by eIF4E as determined by ribonuclease footprinting. These findings indicate that aptamers can achieve high affinity to a specific target protein via global conformational recognition. The genetic mutation and affinity study of variant eIF4E proteins suggests that aptamer 1 binds to eIF4E adjacent to the entrance of the cap-binding slot and blocks the cap-binding pocket, thereby inhibiting translation initiation.
Figures
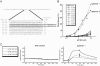
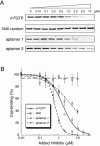
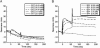
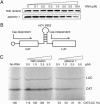
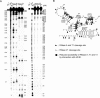
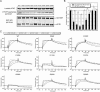
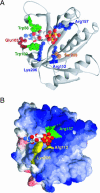
Similar articles
-
Biophysical studies of eIF4E cap-binding protein: recognition of mRNA 5' cap structure and synthetic fragments of eIF4G and 4E-BP1 proteins.J Mol Biol. 2002 Jun 7;319(3):615-35. doi: 10.1016/S0022-2836(02)00328-5. J Mol Biol. 2002. PMID: 12054859
-
Repressor binding to a dorsal regulatory site traps human eIF4E in a high cap-affinity state.EMBO J. 1999 Jul 15;18(14):4068-75. doi: 10.1093/emboj/18.14.4068. EMBO J. 1999. PMID: 10406811 Free PMC article.
-
RNA aptamers to mammalian initiation factor 4G inhibit cap-dependent translation by blocking the formation of initiation factor complexes.RNA. 2006 Oct;12(10):1825-34. doi: 10.1261/rna.2169406. Epub 2006 Aug 29. RNA. 2006. PMID: 16940549 Free PMC article.
-
Regulation of cap-dependent translation by eIF4E inhibitory proteins.Nature. 2005 Feb 3;433(7025):477-80. doi: 10.1038/nature03205. Nature. 2005. PMID: 15690031 Review.
-
eIF4E, the mRNA cap-binding protein: from basic discovery to translational research.Biochem Cell Biol. 2008 Apr;86(2):178-83. doi: 10.1139/O08-034. Biochem Cell Biol. 2008. PMID: 18443631 Review.
Cited by
-
DNA aptamers selectively target Leishmania infantum H2A protein.PLoS One. 2013 Oct 21;8(10):e78886. doi: 10.1371/journal.pone.0078886. eCollection 2013. PLoS One. 2013. PMID: 24205340 Free PMC article.
-
The Runt domain of AML1 (RUNX1) binds a sequence-conserved RNA motif that mimics a DNA element.RNA. 2013 Jul;19(7):927-36. doi: 10.1261/rna.037879.112. Epub 2013 May 24. RNA. 2013. PMID: 23709277 Free PMC article.
-
Major source of antigenic peptides for the MHC class I pathway is produced during the pioneer round of mRNA translation.Proc Natl Acad Sci U S A. 2011 Jul 12;108(28):11572-7. doi: 10.1073/pnas.1104104108. Epub 2011 Jun 27. Proc Natl Acad Sci U S A. 2011. PMID: 21709220 Free PMC article.
-
Dual modulation of Ras-Mnk and PI3K-AKT-mTOR pathways: A Novel c-FLIP inhibitory mechanism of 3-AWA mediated translational attenuation through dephosphorylation of eIF4E.Sci Rep. 2016 Jan 5;6:18800. doi: 10.1038/srep18800. Sci Rep. 2016. PMID: 26728896 Free PMC article.
-
RNA plasticity and selectivity applicable to therapeutics and novel biosensor development.Genes Cells. 2012 May;17(5):344-64. doi: 10.1111/j.1365-2443.2012.01596.x. Epub 2012 Apr 4. Genes Cells. 2012. PMID: 22487172 Free PMC article. Review.
References
-
- Altmann, M., Edery, I., Trachsel, H., and Sonenberg, N. 1988. Site-directed mutagenesis of the tryptophan residues in yeast eukaryotic initiation factor 4E; effects on cap binding activity. J. Biol. Chem. 263: 17229–17232. - PubMed
-
- Avdulov, S., Li, S., Michalek, V., Burrichter, D., Peterson, M., Periman, D.M., Manivel, J.C., Sonenberg, N., Yee, D., Bitterman, P.B., et al. 2004. Activation of translation complex eIF4F is essential for the genesis and maintenance of the malignant phenotype in human mammary epithelial cells. Cancer Cell 5: 553–563. - PubMed
-
- Cai, A., Jankowska-Anyszka, M., Centers, A., Chlebicka, L., Stepinski, J., Stolarski, R., Darzynkiewicz, E., and Rhoads, R.E. 1999. Assessment of mRNA cap analogues as inhibitors of in vitro translation. Biochemistry 38: 8538–8547. - PubMed
-
- Clemens, M.J. and Bommer, U.A. 1999. Translational control: The cancer connection. Int. J. Biochem. Cell. Biol. 31: 1–23. - PubMed
Publication types
MeSH terms
Substances
LinkOut - more resources
Full Text Sources
Other Literature Sources
Research Materials
Miscellaneous