A Tel1/MRX-dependent checkpoint inhibits the metaphase-to-anaphase transition after UV irradiation in the absence of Mec1
- PMID: 15542824
- PMCID: PMC529042
- DOI: 10.1128/MCB.24.23.10126-10144.2004
A Tel1/MRX-dependent checkpoint inhibits the metaphase-to-anaphase transition after UV irradiation in the absence of Mec1
Abstract
In Saccharomyces cerevisiae, Mec1/ATR plays a primary role in sensing and transducing checkpoint signals in response to different types of DNA lesions, while the role of the Tel1/ATM kinase in DNA damage checkpoints is not as well defined. We found that UV irradiation in G(1) in the absence of Mec1 activates a Tel1/MRX-dependent checkpoint, which specifically inhibits the metaphase-to-anaphase transition. Activation of this checkpoint leads to phosphorylation of the downstream checkpoint kinases Rad53 and Chk1, which are required for Tel1-dependent cell cycle arrest, and their adaptor Rad9. The spindle assembly checkpoint protein Mad2 also partially contributes to the G(2)/M arrest of UV-irradiated mec1Delta cells independently of Rad53 phosphorylation and activation. The inability of UV-irradiated mec1Delta cells to undergo anaphase can be relieved by eliminating the anaphase inhibitor Pds1, whose phosphorylation and stabilization in these cells depend on Tel1, suggesting that Pds1 persistence may be responsible for the inability to undergo anaphase. Moreover, while UV irradiation can trigger Mec1-dependent Rad53 phosphorylation and activation in G(1)- and G(2)-arrested cells, Tel1-dependent checkpoint activation requires entry into S phase independently of the cell cycle phase at which cells are UV irradiated, and it is decreased when single-stranded DNA signaling is affected by the rfa1-t11 allele. This indicates that UV-damaged DNA molecules need to undergo structural changes in order to activate the Tel1-dependent checkpoint. Active Clb-cyclin-dependent kinase 1 (CDK1) complexes also participate in triggering this checkpoint and are required to maintain both Mec1- and Tel1-dependent Rad53 phosphorylation, suggesting that they may provide critical phosphorylation events in the DNA damage checkpoint cascade.
Figures
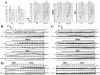
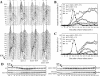
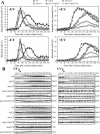
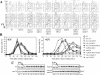
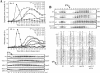
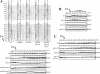
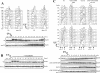
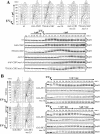
Similar articles
-
Two distinct pathways for inhibiting pds1 ubiquitination in response to DNA damage.J Biol Chem. 2003 Nov 7;278(45):45027-33. doi: 10.1074/jbc.M306783200. Epub 2003 Aug 28. J Biol Chem. 2003. PMID: 12947083
-
Hyperactivation of the yeast DNA damage checkpoint by TEL1 and DDC2 overexpression.EMBO J. 2001 Nov 15;20(22):6485-98. doi: 10.1093/emboj/20.22.6485. EMBO J. 2001. PMID: 11707419 Free PMC article.
-
Control of the DNA damage checkpoint by chk1 and rad53 protein kinases through distinct mechanisms.Science. 1999 Nov 5;286(5442):1166-71. doi: 10.1126/science.286.5442.1166. Science. 1999. PMID: 10550056
-
Stopped for repairs: a new role for nutrient sensing pathways?Cell Cycle. 2004 Jul;3(7):865-8. Epub 2004 Jul 17. Cell Cycle. 2004. PMID: 15190205 Review.
-
Interplays between ATM/Tel1 and ATR/Mec1 in sensing and signaling DNA double-strand breaks.DNA Repair (Amst). 2013 Oct;12(10):791-9. doi: 10.1016/j.dnarep.2013.07.009. Epub 2013 Aug 13. DNA Repair (Amst). 2013. PMID: 23953933 Review.
Cited by
-
Tel1/ATM Signaling to the Checkpoint Contributes to Replicative Senescence in the Absence of Telomerase.Genetics. 2019 Oct;213(2):411-429. doi: 10.1534/genetics.119.302391. Epub 2019 Aug 7. Genetics. 2019. PMID: 31391264 Free PMC article.
-
Replication and recombination factors contributing to recombination-dependent bypass of DNA lesions by template switch.PLoS Genet. 2010 Nov 11;6(11):e1001205. doi: 10.1371/journal.pgen.1001205. PLoS Genet. 2010. PMID: 21085632 Free PMC article.
-
γH2A/γH2AX Mediates DNA Damage-Specific Control of Checkpoint Signaling in Saccharomyces cerevisiae.Int J Mol Sci. 2024 Feb 20;25(5):2462. doi: 10.3390/ijms25052462. Int J Mol Sci. 2024. PMID: 38473708 Free PMC article.
-
Dephosphorylation of gamma H2A by Glc7/protein phosphatase 1 promotes recovery from inhibition of DNA replication.Mol Cell Biol. 2010 Jan;30(1):131-45. doi: 10.1128/MCB.01000-09. Mol Cell Biol. 2010. PMID: 19884341 Free PMC article.
-
Colocalization of sensors is sufficient to activate the DNA damage checkpoint in the absence of damage.Mol Cell. 2008 May 9;30(3):267-76. doi: 10.1016/j.molcel.2008.03.023. Mol Cell. 2008. PMID: 18471973 Free PMC article.
References
-
- Agarwal, R., Z. Tang, H. Yu, and O. Cohen-Fix. 2003. Two distinct pathways for inhibiting Pds1 ubiquitination in response to DNA damage. J. Biol. Chem. 278:45027-45033. - PubMed
-
- Andegeko, Y., L. Moyal, L. Mittelman, I. Tsarfaty, Y. Shiloh, and G. Rotman. 2001. Nuclear retention of ATM at sites of DNA double strand breaks. J. Biol. Chem. 276:38224-38230. - PubMed
-
- Bakkenist, C. J., and M. B. Kastan. 2003. DNA damage activates ATM through intermolecular autophosphorylation and dimer dissociation. Nature 421:499-506. - PubMed
-
- Banin, S., L. Moyal, S. Shieh, Y. Taya, C. W. Anderson, L. Chessa, N. I. Smorodinsky, C. Prives, Y. Reiss, Y. Shiloh, and Y. Ziv. 1998. Enhanced phosphorylation of p53 by ATM in response to DNA damage. Science 281:1674-1677. - PubMed
Publication types
MeSH terms
Substances
LinkOut - more resources
Full Text Sources
Molecular Biology Databases
Research Materials
Miscellaneous