Conformational defects slow Golgi exit, block oligomerization, and reduce raft affinity of caveolin-1 mutant proteins
- PMID: 15304521
- PMCID: PMC519149
- DOI: 10.1091/mbc.e04-06-0480
Conformational defects slow Golgi exit, block oligomerization, and reduce raft affinity of caveolin-1 mutant proteins
Abstract
Caveolin-1, a structural protein of caveolae, is cleared unusually slowly from the Golgi apparatus during biosynthetic transport. Furthermore, several caveolin-1 mutant proteins accumulate in the Golgi apparatus. We examined this behavior further in this mutant study. Golgi accumulation probably resulted from loss of Golgi exit information, not exposure of cryptic retention signals, because several deletion mutants accumulated in the Golgi apparatus. Alterations throughout the protein caused Golgi accumulation. Thus, most probably acted indirectly, by affecting overall conformation, rather than by disrupting specific Golgi exit motifs. Consistent with this idea, almost all the Golgi-localized mutant proteins failed to oligomerize normally (even with an intact oligomerization domain), and they showed reduced raft affinity in an in vitro detergent-insolubility assay. A few mutant proteins formed unstable oligomers that migrated unusually slowly on blue native gels. Only one mutant protein, which lacked the first half of the N-terminal hydrophilic domain, accumulated in the Golgi apparatus despite normal oligomerization and raft association. These results suggested that transport of caveolin-1 through the Golgi apparatus is unusually difficult. The conformation of caveolin-1 may be optimized to overcome this difficulty, but remain very sensitive to mutation. Disrupting conformation can coordinately affect oligomerization, raft affinity, and Golgi exit of caveolin-1.
Figures
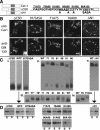
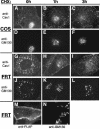
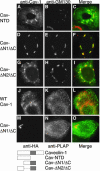
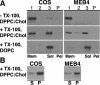
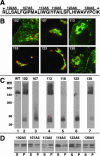
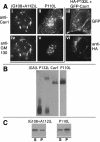
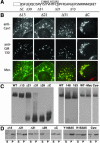
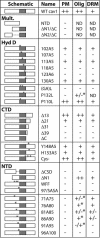
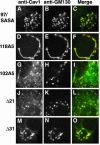
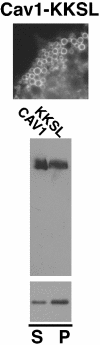
Similar articles
-
The scaffolding domain of caveolin 2 is responsible for its Golgi localization in Caco-2 cells.J Cell Sci. 2002 Dec 1;115(Pt 23):4457-67. doi: 10.1242/jcs.00130. J Cell Sci. 2002. PMID: 12414992
-
Tuberin is a component of lipid rafts and mediates caveolin-1 localization: role of TSC2 in post-Golgi transport.Exp Cell Res. 2004 May 1;295(2):512-24. doi: 10.1016/j.yexcr.2004.01.022. Exp Cell Res. 2004. PMID: 15093748
-
Accumulation of caveolin in the endoplasmic reticulum redirects the protein to lipid storage droplets.J Cell Biol. 2001 Mar 5;152(5):1071-8. doi: 10.1083/jcb.152.5.1071. J Cell Biol. 2001. PMID: 11238461 Free PMC article.
-
Caveolins: structure and function in signal transduction.Cell Mol Biol Lett. 2004;9(2):195-220. Cell Mol Biol Lett. 2004. PMID: 15213803 Review.
-
VIP21-Caveolin, a protein of the trans-Golgi network and caveolae.FEBS Lett. 1994 Jun 6;346(1):88-91. doi: 10.1016/0014-5793(94)00466-8. FEBS Lett. 1994. PMID: 8206165 Review.
Cited by
-
Renal Na+-K+-Cl- cotransporter activity and vasopressin-induced trafficking are lipid raft-dependent.Am J Physiol Renal Physiol. 2008 Sep;295(3):F789-802. doi: 10.1152/ajprenal.90227.2008. Epub 2008 Jun 25. Am J Physiol Renal Physiol. 2008. PMID: 18579701 Free PMC article.
-
Caveolin-1 is an aggresome-inducing protein.Sci Rep. 2016 Dec 8;6:38681. doi: 10.1038/srep38681. Sci Rep. 2016. PMID: 27929047 Free PMC article.
-
Aberrant caveolin-1-mediated Smad signaling and proliferation identified by analysis of adenine 474 deletion mutation (c.474delA) in patient fibroblasts: a new perspective on the mechanism of pulmonary hypertension.Mol Biol Cell. 2017 May 1;28(9):1177-1185. doi: 10.1091/mbc.E16-11-0790. Mol Biol Cell. 2017. PMID: 28468941 Free PMC article.
-
Tagging strategies strongly affect the fate of overexpressed caveolin-1.Traffic. 2015 Apr;16(4):417-38. doi: 10.1111/tra.12254. Traffic. 2015. PMID: 25639341 Free PMC article.
-
Assembly and Turnover of Caveolae: What Do We Really Know?Front Cell Dev Biol. 2016 Jun 27;4:68. doi: 10.3389/fcell.2016.00068. eCollection 2016. Front Cell Dev Biol. 2016. PMID: 27446919 Free PMC article. Review.
References
-
- Arbuzova, A., Wang, L., Wang, J., Hangyas-Mihalyne, G., Murray, D., Honig, B., and McLaughlin, S. (2000). Membrane binding of peptides containing both basic and aromatic residues. Experimental studies with peptides corresponding to the scaffolding region of caveolin and the effector region of MARCKS. Biochemistry 39, 10330-10339. - PubMed
-
- Arreaza, G., and Brown, D.A. (1995). Sorting and intracellular trafficking of a glycosylphosphatidylinositol-anchored protein and two hybrid proteins with the same ectodomain in MDCK kidney epithelial cells. J. Biol. Chem. 270, 23641-23647. - PubMed
-
- Bretscher, M.S., and Munro, S. (1993). Cholesterol and the Golgi apparatus. Science 261, 1280-1281. - PubMed
-
- Breuza, L., Corby, S., Arsanto, J.-P., Delgrossi, M.-H., Scheiffele, P., and Le Bivic, A. (2002). The scaffolding domain of caveolin 2 is responsible for its Golgi localization in Caco-2 cells. J. Cell Sci. 115, 4457-4467. - PubMed
-
- Brown, D.A., and London, E. (2000). Structure and function of sphingolipid- and cholesterol-rich membrane rafts. J. Biol. Chem. 275, 17221-17224. - PubMed
Publication types
MeSH terms
Substances
Grants and funding
LinkOut - more resources
Full Text Sources
Molecular Biology Databases