Ability of the human cytomegalovirus IE1 protein to modulate sumoylation of PML correlates with its functional activities in transcriptional regulation and infectivity in cultured fibroblast cells
- PMID: 15163746
- PMCID: PMC416510
- DOI: 10.1128/JVI.78.12.6527-6542.2004
Ability of the human cytomegalovirus IE1 protein to modulate sumoylation of PML correlates with its functional activities in transcriptional regulation and infectivity in cultured fibroblast cells
Abstract
In one of the earliest events in human cytomegalovirus (HCMV)-infected cells, the major immediate-early (IE) protein IE1 initially targets to and then disrupts the nuclear structures known as PML oncogenic domains (PODs) or nuclear domain 10. Recent studies have suggested that modification of PML by SUMO is essential to form PODs and that IE1 both binds to PML and may disrupt PODs by preventing or removing SUMO adducts on PML. In this study, we showed that in contrast to herpes simplex virus type 1 (HSV-1) IE110 (ICP0), the loss of sumoylated forms of PML by cotransfected IE1 was resistant to the proteasome inhibitor MG132 and that IE1 did not reduce the level of unmodified PML. Reduced sumoylation of PML was also observed in U373 cells after infection with wild-type HCMV and proved to require IE1 protein expression. Mutational analysis revealed that the central hydrophobic domain of IE1, including Leu174, is required for both PML binding and loss of PML sumoylation and confirmed that all IE1 mutants tested that were deficient in these functions also failed both to target to PODs and to disrupt PODs. These same mutants were also inactive in several reporter gene transactivation assays and in inhibition of PML-mediated repression. Importantly, a viral DNA genome containing an IE1 gene with a deletion [IE1(Delta290-320)] that was defective in these activities was not infectious when transfected into permissive fibroblast cells, but the mutant IE1(K450R), which is defective in IE1 sumoylation, remained infectious. Our mutational analysis strengthens the idea that interference by IE1 with both the sumoylation of PML and its repressor activity requires a physical interaction with PML that also leads to disruption of PODs. These activities of IE1 also correlate with several unusual transcriptional transactivation functions of IE1 and may be requirements for efficient initiation of the lytic cycle in vivo.
Figures
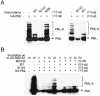
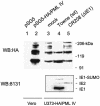
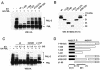
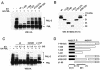
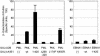
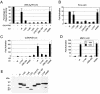
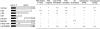
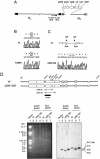
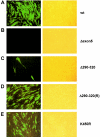
Similar articles
-
Proteasome-independent disruption of PML oncogenic domains (PODs), but not covalent modification by SUMO-1, is required for human cytomegalovirus immediate-early protein IE1 to inhibit PML-mediated transcriptional repression.J Virol. 2001 Nov;75(22):10683-95. doi: 10.1128/JVI.75.22.10683-10695.2001. J Virol. 2001. PMID: 11602710 Free PMC article.
-
Disruption of PML-associated nuclear bodies by IE1 correlates with efficient early stages of viral gene expression and DNA replication in human cytomegalovirus infection.Virology. 2000 Aug 15;274(1):39-55. doi: 10.1006/viro.2000.0448. Virology. 2000. PMID: 10936087
-
The ND10 Component Promyelocytic Leukemia Protein Acts as an E3 Ligase for SUMOylation of the Major Immediate Early Protein IE1 of Human Cytomegalovirus.J Virol. 2017 Apr 28;91(10):e02335-16. doi: 10.1128/JVI.02335-16. Print 2017 May 15. J Virol. 2017. PMID: 28250117 Free PMC article.
-
The Human CMV IE1 Protein: An Offender of PML Nuclear Bodies.Adv Anat Embryol Cell Biol. 2017;223:77-94. doi: 10.1007/978-3-319-53168-7_4. Adv Anat Embryol Cell Biol. 2017. PMID: 28528440 Review.
-
PML and the oncogenic nuclear domains in regulating transcriptional repression.Curr Opin Cell Biol. 2000 Oct;12(5):641-4. doi: 10.1016/s0955-0674(00)00144-7. Curr Opin Cell Biol. 2000. PMID: 10978902 Review.
Cited by
-
Promyelocytic leukemia-nuclear body proteins: herpesvirus enemies, accomplices, or both?Future Virol. 2008 May 1;3(3):265-277. doi: 10.2217/17460794.3.3.265. Future Virol. 2008. PMID: 19763230 Free PMC article.
-
Cytomegalovirus immediate-early 1 proteins form a structurally distinct protein class with adaptations determining cross-species barriers.PLoS Pathog. 2021 Aug 9;17(8):e1009863. doi: 10.1371/journal.ppat.1009863. eCollection 2021 Aug. PLoS Pathog. 2021. PMID: 34370791 Free PMC article.
-
Interplay between viruses and host sumoylation pathways.Nat Rev Microbiol. 2013 Jun;11(6):400-11. doi: 10.1038/nrmicro3015. Epub 2013 Apr 29. Nat Rev Microbiol. 2013. PMID: 23624814 Review.
-
PML Body Component Sp100A Is a Cytosolic Responder to IFN and Activator of Antiviral ISGs.mBio. 2022 Dec 20;13(6):e0204422. doi: 10.1128/mbio.02044-22. Epub 2022 Nov 16. mBio. 2022. PMID: 36383022 Free PMC article.
-
A Virally Encoded DeSUMOylase Activity Is Required for Cytomegalovirus Reactivation from Latency.Cell Rep. 2018 Jul 17;24(3):594-606. doi: 10.1016/j.celrep.2018.06.048. Cell Rep. 2018. PMID: 30021158 Free PMC article.
References
-
- Ahn, J. H., E. J. Brignole III, and G. S. Hayward. 1998. Disruption of PML subnuclear domains by the acidic IE1 protein of human cytomegalovirus is mediated through interaction with PML and may modulate a RING finger-dependent cryptic transactivator function of PML. Mol. Cell. Biol 18:4899-4913. - PMC - PubMed
-
- Ahn, J. H., and G. S. Hayward. 2000. Disruption of PML-associated nuclear bodies by IE1 correlates with efficient early stages of viral gene expression and DNA replication in human cytomegalovirus infection. Virology 274:39-55. - PubMed
-
- Ahn, J. H., W. J. Jang, and G. S. Hayward. 1999. The human cytomegalovirus IE2 and UL112-113 proteins accumulate in viral DNA replication compartments that initiate from the periphery of promyelocytic leukemia protein-associated nuclear bodies (PODs or ND10). J. Virol. 73:10458-10471. - PMC - PubMed
Publication types
MeSH terms
Substances
Grants and funding
LinkOut - more resources
Full Text Sources
Other Literature Sources
Molecular Biology Databases