Molecular surface of tarantula toxins interacting with voltage sensors in K(v) channels
- PMID: 15051809
- PMCID: PMC2217462
- DOI: 10.1085/jgp.200309005
Molecular surface of tarantula toxins interacting with voltage sensors in K(v) channels
Abstract
The venom from spiders, scorpions, and sea anemone contain a rich diversity of protein toxins that interact with ion channel voltage sensors. Although atomic structures have been solved for many of these toxins, the surfaces that are critical for interacting with voltage sensors are poorly defined. Hanatoxin and SGTx are tarantula toxins that inhibit activation of K(v) channels by interacting with each of the four voltage sensors. In this study we set out to identify the active surface of these toxins by alanine-scanning SGTx and characterizing the interaction of each mutant with the K(v)2.1 channel. Examination of the concentration dependence for inhibition identified 15 mutants with little effect on the concentration dependence for toxin inhibition of the K(v)2.1 channel, and 11 mutants that display moderate to dramatic perturbations. Mapping of these results onto the structure of SGTx identifies one face of the toxin where mutations with pronounced perturbations cluster together, and a backside of the toxin where mutations are well tolerated. The active surface of SGTx contains a ring-like assembly of highly polar residues, with two basic residues that are particularly critical, concentrically arranged around a hydrophobic protrusion containing critical aliphatic and aromatic residues. These results identify the active surface of the toxin and reveal the types of side chains that are important for interacting with voltage sensors.
Figures
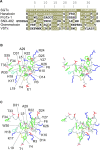
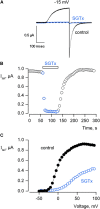
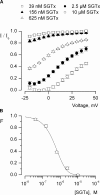
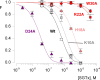
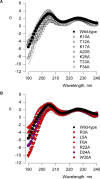
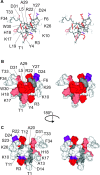
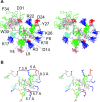
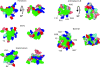
Similar articles
-
Analysis of the interaction of tarantula toxin Jingzhaotoxin-III (β-TRTX-Cj1α) with the voltage sensor of Kv2.1 uncovers the molecular basis for cross-activities on Kv2.1 and Nav1.5 channels.Biochemistry. 2013 Oct 22;52(42):7439-48. doi: 10.1021/bi4006418. Epub 2013 Oct 7. Biochemistry. 2013. PMID: 24044413
-
Tarantula toxins interacting with voltage sensors in potassium channels.Toxicon. 2007 Feb;49(2):213-30. doi: 10.1016/j.toxicon.2006.09.024. Epub 2006 Sep 29. Toxicon. 2007. PMID: 17097703 Free PMC article. Review.
-
Tarantula toxins use common surfaces for interacting with Kv and ASIC ion channels.Elife. 2015 May 7;4:e06774. doi: 10.7554/eLife.06774. Elife. 2015. PMID: 25948544 Free PMC article.
-
Molecular Interactions between Tarantula Toxins and Low-Voltage-Activated Calcium Channels.Sci Rep. 2016 Apr 5;6:23894. doi: 10.1038/srep23894. Sci Rep. 2016. PMID: 27045173 Free PMC article.
-
Ion-channel modulators: more diversity than previously thought.Chembiochem. 2011 Aug 16;12(12):1808-12. doi: 10.1002/cbic.201100236. Epub 2011 Jul 1. Chembiochem. 2011. PMID: 21726033 Review.
Cited by
-
Molecular dynamics simulations of a stretch-activated channel inhibitor GsMTx4 with lipid membranes: two binding modes and effects of lipid structure.Biophys J. 2007 Jun 15;92(12):4233-43. doi: 10.1529/biophysj.106.101071. Epub 2007 Mar 23. Biophys J. 2007. PMID: 17384064 Free PMC article.
-
Structural interactions of a voltage sensor toxin with lipid membranes.Proc Natl Acad Sci U S A. 2014 Dec 16;111(50):E5463-70. doi: 10.1073/pnas.1415324111. Epub 2014 Dec 1. Proc Natl Acad Sci U S A. 2014. PMID: 25453087 Free PMC article.
-
Modulatory features of the novel spider toxin μ-TRTX-Df1a isolated from the venom of the spider Davus fasciatus.Br J Pharmacol. 2017 Aug;174(15):2528-2544. doi: 10.1111/bph.13865. Epub 2017 Jun 27. Br J Pharmacol. 2017. PMID: 28542706 Free PMC article.
-
Gating modifier toxins isolated from spider venom: Modulation of voltage-gated sodium channels and the role of lipid membranes.J Biol Chem. 2018 Jun 8;293(23):9041-9052. doi: 10.1074/jbc.RA118.002553. Epub 2018 Apr 27. J Biol Chem. 2018. PMID: 29703751 Free PMC article.
-
Chemical Synthesis and NMR Solution Structure of Conotoxin GXIA from Conus geographus.Mar Drugs. 2021 Jan 26;19(2):60. doi: 10.3390/md19020060. Mar Drugs. 2021. PMID: 33530397 Free PMC article.