Modification of de novo DNA methyltransferase 3a (Dnmt3a) by SUMO-1 modulates its interaction with histone deacetylases (HDACs) and its capacity to repress transcription
- PMID: 14752048
- PMCID: PMC373322
- DOI: 10.1093/nar/gkh195
Modification of de novo DNA methyltransferase 3a (Dnmt3a) by SUMO-1 modulates its interaction with histone deacetylases (HDACs) and its capacity to repress transcription
Abstract
The de novo DNA methyltransferase Dnmt3a is one of three mammalian DNA methyltransferases that has been shown to play crucial roles in embryonic development, genomic imprinting and transcriptional silencing. Despite its importance, very little is known about how the enzymatic activity and transcriptional repression functions of Dnmt3a are regulated. Here we show that Dnmt3a interacts with multiple components of the sumoylation machinery, namely the E2 sumo conjugating enzyme Ubc9 and the E3 sumo ligases PIAS1 and PIASxalpha, all of which are involved in conjugating the small ubiquitin-like modifier polypeptide, SUMO-1, to its target proteins. Dnmt3a is modified by SUMO-1 in vivo and in vitro and the region of Dnmt3a responsible for interaction maps to the N-terminal regulatory domain. Functionally, sumoylation of Dnmt3a disrupts its ability to interact with histone deacetylases (HDAC1/2), but not with another interaction partner, Dnmt3b. Conditions that enhance the sumoylation of Dnmt3a in vivo abolish its capacity to repress transcription. These studies reveal a new level of regulation governing Dnmt3a whereby a post-translational modification can dramatically regulate its interaction with specific protein partners and alter its ability to repress transcription.
Figures
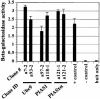
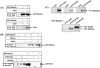
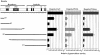
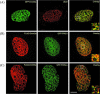
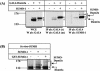
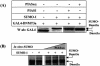
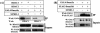
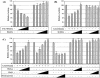
Similar articles
-
Polycomb protein Cbx4 promotes SUMO modification of de novo DNA methyltransferase Dnmt3a.Biochem J. 2007 Jul 15;405(2):369-78. doi: 10.1042/BJ20061873. Biochem J. 2007. PMID: 17439403 Free PMC article.
-
Dnmt3b, de novo DNA methyltransferase, interacts with SUMO-1 and Ubc9 through its N-terminal region and is subject to modification by SUMO-1.Biochem Biophys Res Commun. 2001 Dec 14;289(4):862-8. doi: 10.1006/bbrc.2001.6057. Biochem Biophys Res Commun. 2001. PMID: 11735126
-
SUMOylation of the corepressor N-CoR modulates its capacity to repress transcription.Mol Biol Cell. 2006 Apr;17(4):1643-51. doi: 10.1091/mbc.e05-07-0610. Epub 2006 Jan 18. Mol Biol Cell. 2006. PMID: 16421255 Free PMC article.
-
Something about SUMO inhibits transcription.Curr Opin Genet Dev. 2005 Oct;15(5):536-41. doi: 10.1016/j.gde.2005.07.004. Curr Opin Genet Dev. 2005. PMID: 16095902 Review.
-
SUMO: getting it on.Biochem Soc Trans. 2007 Dec;35(Pt 6):1409-13. doi: 10.1042/BST0351409. Biochem Soc Trans. 2007. PMID: 18031233 Review.
Cited by
-
Stress-induced gene expression and behavior are controlled by DNA methylation and methyl donor availability in the dentate gyrus.Proc Natl Acad Sci U S A. 2016 Apr 26;113(17):4830-5. doi: 10.1073/pnas.1524857113. Epub 2016 Apr 12. Proc Natl Acad Sci U S A. 2016. PMID: 27078100 Free PMC article.
-
PPARgamma1 and LXRalpha face a new regulator of macrophage cholesterol homeostasis and inflammatory responsiveness, AEBP1.Nucl Recept Signal. 2010 Apr 16;8:e004. doi: 10.1621/nrs.08004. Nucl Recept Signal. 2010. PMID: 20419060 Free PMC article. Review.
-
Identification of intracellular proteins and signaling pathways in human endothelial cells regulated by angiotensin-(1-7).J Proteomics. 2016 Jan 1;130:129-39. doi: 10.1016/j.jprot.2015.09.020. Epub 2015 Sep 24. J Proteomics. 2016. PMID: 26388433 Free PMC article.
-
Roles of pRB in the Regulation of Nucleosome and Chromatin Structures.Biomed Res Int. 2016;2016:5959721. doi: 10.1155/2016/5959721. Epub 2016 Dec 22. Biomed Res Int. 2016. PMID: 28101510 Free PMC article. Review.
-
Talking to chromatin: post-translational modulation of polycomb group function.Epigenetics Chromatin. 2009 Sep 1;2(1):10. doi: 10.1186/1756-8935-2-10. Epigenetics Chromatin. 2009. PMID: 19723311 Free PMC article.
References
-
- Robertson K.D. (2002) DNA methylation and chromatin—unraveling the tangled web. Oncogene, 21, 5361–5379. - PubMed
-
- Baylin S.B., Esteller,M., Rountree,M.R., Bachman,K.E., Schuebel,K. and Herman,J.G. (2001) Aberrant patterns of DNA methylation, chromatin formation and gene expression in cancer. Hum. Mol. Genet., 10, 687–692. - PubMed
-
- Jones P.A. and Baylin,S.B. (2002) The fundamental role of epigenetic events in cancer. Nature Rev. Genet., 3, 415–428. - PubMed
-
- Bestor T.H. (2000) The DNA methyltransferases. Hum. Mol. Genet., 9, 2395–2402. - PubMed
-
- Li E. (2002) Chromatin modification and epigenetic reprogramming in mammalian development. Nature Rev. Genet., 3, 662–673. - PubMed
Publication types
MeSH terms
Substances
LinkOut - more resources
Full Text Sources
Other Literature Sources
Molecular Biology Databases
Miscellaneous