The Rep protein of adeno-associated virus type 2 interacts with single-stranded DNA-binding proteins that enhance viral replication
- PMID: 14671124
- PMCID: PMC303412
- DOI: 10.1128/jvi.78.1.441-453.2004
The Rep protein of adeno-associated virus type 2 interacts with single-stranded DNA-binding proteins that enhance viral replication
Abstract
Adeno-associated virus (AAV) type 2 is a human parvovirus whose replication is dependent upon cellular proteins as well as functions supplied by helper viruses. The minimal herpes simplex virus type 1 (HSV-1) proteins that support AAV replication in cell culture are the helicase-primase complex of UL5, UL8, and UL52, together with the UL29 gene product ICP8. We show that AAV and HSV-1 replication proteins colocalize at discrete intranuclear sites. Transfections with mutant genes demonstrate that enzymatic functions of the helicase-primase are not essential. The ICP8 protein alone enhances AAV replication in an in vitro assay. We also show localization of the cellular replication protein A (RPA) at AAV centers under a variety of conditions that support replication. In vitro assays demonstrate that the AAV Rep68 and Rep78 proteins interact with the single-stranded DNA-binding proteins (ssDBPs) of Ad (Ad-DBP), HSV-1 (ICP8), and the cell (RPA) and that these proteins enhance binding and nicking of Rep proteins at the origin. These results highlight the importance of intranuclear localization and suggest that Rep interaction with multiple ssDBPs allows AAV to replicate under a diverse set of conditions.
Figures
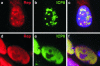
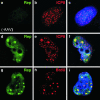
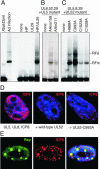
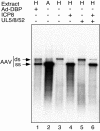
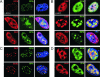
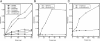
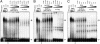
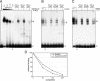
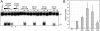
Similar articles
-
Live covisualization of competing adeno-associated virus and herpes simplex virus type 1 DNA replication: molecular mechanisms of interaction.J Virol. 2007 May;81(9):4732-43. doi: 10.1128/JVI.02476-06. Epub 2007 Feb 21. J Virol. 2007. PMID: 17314170 Free PMC article.
-
Definition of herpes simplex virus type 1 helper activities for adeno-associated virus early replication events.PLoS Pathog. 2009 Mar;5(3):e1000340. doi: 10.1371/journal.ppat.1000340. Epub 2009 Mar 13. PLoS Pathog. 2009. PMID: 19282980 Free PMC article.
-
Role of the herpes simplex virus helicase-primase complex during adeno-associated virus DNA replication.J Virol. 2006 Jun;80(11):5241-50. doi: 10.1128/JVI.02718-05. J Virol. 2006. PMID: 16699004 Free PMC article.
-
HSV-1 DNA Replication-Coordinated Regulation by Viral and Cellular Factors.Viruses. 2021 Oct 7;13(10):2015. doi: 10.3390/v13102015. Viruses. 2021. PMID: 34696446 Free PMC article. Review.
-
Replication and recombination of herpes simplex virus DNA.J Biol Chem. 2011 May 6;286(18):15619-24. doi: 10.1074/jbc.R111.233981. Epub 2011 Mar 1. J Biol Chem. 2011. PMID: 21362621 Free PMC article. Review.
Cited by
-
Targeting human papillomavirus genome replication for antiviral drug discovery.Antivir Ther. 2013;18(3):271-83. doi: 10.3851/IMP2612. Epub 2013 Apr 24. Antivir Ther. 2013. PMID: 23615820 Free PMC article. Review.
-
Regulation of adeno-associated virus DNA replication by the cellular TAF-I/set complex.J Virol. 2006 Jul;80(14):6855-64. doi: 10.1128/JVI.00383-06. J Virol. 2006. PMID: 16809291 Free PMC article.
-
Recent Advances in Molecular Biology of Human Bocavirus 1 and Its Applications.Front Microbiol. 2021 Jun 16;12:696604. doi: 10.3389/fmicb.2021.696604. eCollection 2021. Front Microbiol. 2021. PMID: 34220786 Free PMC article. Review.
-
Fluorescence Microscopy in Adeno-Associated Virus Research.Viruses. 2023 May 16;15(5):1174. doi: 10.3390/v15051174. Viruses. 2023. PMID: 37243260 Free PMC article. Review.
-
Recombinant adeno-associated viral vectors are deficient in provoking a DNA damage response.J Virol. 2008 Aug;82(15):7379-87. doi: 10.1128/JVI.00358-08. Epub 2008 May 7. J Virol. 2008. PMID: 18463154 Free PMC article.
References
-
- Arana, M. E., B. Haq, N. Tanguy Le Gac, and P. E. Boehmer. 2001. Modulation of the herpes simplex virus type-1 UL9 DNA helicase by its cognate single-strand DNA-binding protein, ICP8. J. Biol. Chem. 276:6840-6845. - PubMed
-
- Biswas, N., and S. K. Weller. 1999. A mutation in the C-terminal putative Zn2+ finger motif of UL52 severely affects the biochemical activities of the HSV-1 helicase-primase subcomplex. J. Biol. Chem. 274:8068-8076. - PubMed
-
- Biswas, N., and S. K. Weller. 2001. The UL5 and UL52 subunits of the herpes simplex virus type 1 helicase-primase subcomplex exhibit a complex interdependence for DNA binding. J. Biol. Chem. 276:17610-17619. - PubMed
-
- Boehmer, P. E. 1998. The herpes simplex virus type-1 single-strand DNA-binding protein, ICP8, increases the processivity of the UL9 protein DNA helicase. J. Biol. Chem. 273:2676-2683. - PubMed
Publication types
MeSH terms
Substances
Grants and funding
LinkOut - more resources
Full Text Sources