Anoxic fibroblasts activate a replication checkpoint that is bypassed by E1a
- PMID: 14645516
- PMCID: PMC309642
- DOI: 10.1128/MCB.23.24.9032-9045.2003
Anoxic fibroblasts activate a replication checkpoint that is bypassed by E1a
Abstract
Little is known about cell cycle regulation in hypoxic cells, despite its significance. We utilized an experimentally tractable model to study the proliferative responses of rat fibroblasts when rendered hypoxic (0.5% oxygen) or anoxic (<0.01% oxygen). Hypoxic cells underwent G1 arrest, whereas anoxic cells also demonstrated S-phase arrest due to suppression of DNA initiation. Upon reoxygenation, only those cells arrested in G1 were able to resume proliferation. The oncoprotein E1a induced p53-independent apoptosis in anoxic cells, which when suppressed by Bcl-2 permitted proliferation despite anoxia. E1a expression led to marked increases in the transcription factor E2F, and overexpression of E2F-1 allowed proliferation in hypoxic cells, although it had minimal effect on the anoxic suppression of DNA initiation. We thus demonstrate two distinct cell cycle responses to low oxygen and suggest that alterations that lead to increased E2F can overcome hypoxic G1 arrest but that additional alterations, promoted by E1a expression, are necessary for neoplastic cells to proliferate despite anoxia.
Figures
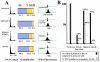
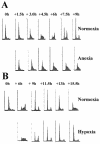
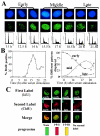
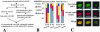
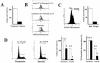
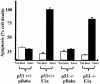
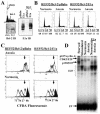
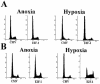
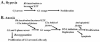
Similar articles
-
[Changes in the activity of cyclin-kinase complexes governing cell transition from G1 phase to DNA replication phase in E1A + c-Ha-ras transformants transfected with the bcl-2 gene].Tsitologiia. 2003;45(2):149-57. Tsitologiia. 2003. PMID: 12722479 Russian.
-
[E1A oncogene effect on the ability of p21(Waf1) to regulate G1/S arrest in E1A-expressing transformants following irradiation].Tsitologiia. 2005;47(12):1063-70. Tsitologiia. 2005. PMID: 16706194 Russian.
-
Deregulation of p53/p21Cip1/Waf1 pathway contributes to polyploidy and apoptosis of E1A+cHa-ras transformed cells after gamma-irradiation.Oncogene. 1999 Oct 7;18(41):5611-9. doi: 10.1038/sj.onc.1202945. Oncogene. 1999. PMID: 10523840
-
Activated H-ras rescues E1A-induced apoptosis and cooperates with E1A to overcome p53-dependent growth arrest.Mol Cell Biol. 1995 Aug;15(8):4536-44. doi: 10.1128/MCB.15.8.4536. Mol Cell Biol. 1995. PMID: 7623844 Free PMC article.
-
Adenovirus and cell cycle control.Front Biosci. 2002 May 1;7:d1369-95. doi: 10.2741/ben. Front Biosci. 2002. PMID: 11991831 Review.
Cited by
-
Epithelial and mesenchymal tumor compartments exhibit in vivo complementary patterns of vascular perfusion and glucose metabolism.Neoplasia. 2007 Nov;9(11):900-8. doi: 10.1593/neo.07541. Neoplasia. 2007. PMID: 18030358 Free PMC article.
-
Tumor hypoxia as a driving force in genetic instability.Genome Integr. 2013 Oct 24;4(1):5. doi: 10.1186/2041-9414-4-5. Genome Integr. 2013. PMID: 24152759 Free PMC article.
-
Hypoxic activation of ATR and the suppression of the initiation of DNA replication through cdc6 degradation.Oncogene. 2012 Sep 6;31(36):4076-84. doi: 10.1038/onc.2011.585. Epub 2011 Dec 19. Oncogene. 2012. PMID: 22179839 Free PMC article.
-
Proliferating fibroblasts at the invading tumour edge of colorectal adenocarcinomas are associated with endogenous markers of hypoxia, acidity, and oxidative stress.J Clin Pathol. 2005 Oct;58(10):1033-8. doi: 10.1136/jcp.2005.026260. J Clin Pathol. 2005. PMID: 16189147 Free PMC article.
-
ATM activation and signaling under hypoxic conditions.Mol Cell Biol. 2009 Jan;29(2):526-37. doi: 10.1128/MCB.01301-08. Epub 2008 Nov 3. Mol Cell Biol. 2009. PMID: 18981219 Free PMC article.
References
-
- Angus, S. P., L. J. Wheeler, S. A. Ranmal, X. Zhang, M. P. Markey, C. K. Mathews, and E. S. Knudsen. 2002. Retinoblastoma tumor suppressor targets dNTP metabolism to regulate DNA replication. J. Biol. Chem. 277:44376-44384. - PubMed
Publication types
MeSH terms
Substances
Grants and funding
LinkOut - more resources
Full Text Sources
Research Materials
Miscellaneous