Decatenation of DNA circles by FtsK-dependent Xer site-specific recombination
- PMID: 14633998
- PMCID: PMC291834
- DOI: 10.1093/emboj/cdg589
Decatenation of DNA circles by FtsK-dependent Xer site-specific recombination
Abstract
DNA replication results in interlinked (catenated) sister duplex molecules as a consequence of the intertwined helices that comprise duplex DNA. DNA topoisomerases play key roles in decatenation. We demonstrate a novel, efficient and directional decatenation process in vitro, which uses the combination of the Escherichia coli XerCD site-specific recombination system and a protein, FtsK, which facilitates simple synapsis of dif recombination sites during its translocation along DNA. We propose that the FtsK-XerCD recombination machinery, which converts chromosomal dimers to monomers, may also function in vivo in removing the final catenation links remaining upon completion of DNA replication.
Figures
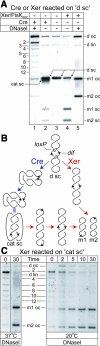
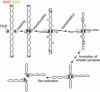
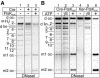
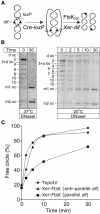
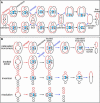
Similar articles
-
Delayed activation of Xer recombination at dif by FtsK during septum assembly in Escherichia coli.Mol Microbiol. 2008 May;68(4):1018-28. doi: 10.1111/j.1365-2958.2008.06212.x. Epub 2008 Mar 19. Mol Microbiol. 2008. PMID: 18363794
-
Extent of the activity domain and possible roles of FtsK in the Escherichia coli chromosome terminus.Mol Microbiol. 2005 Jun;56(6):1539-48. doi: 10.1111/j.1365-2958.2005.04633.x. Mol Microbiol. 2005. PMID: 15916604
-
Unlinking chromosome catenanes in vivo by site-specific recombination.EMBO J. 2007 Oct 3;26(19):4228-38. doi: 10.1038/sj.emboj.7601849. Epub 2007 Sep 6. EMBO J. 2007. PMID: 17805344 Free PMC article.
-
Simple topology: FtsK-directed recombination at the dif site.Biochem Soc Trans. 2013 Apr;41(2):595-600. doi: 10.1042/BST20120299. Biochem Soc Trans. 2013. PMID: 23514160 Review.
-
The Escherichia coli DNA translocase FtsK.Biochem Soc Trans. 2010 Apr;38(2):395-8. doi: 10.1042/BST0380395. Biochem Soc Trans. 2010. PMID: 20298190 Review.
Cited by
-
Functional taxonomy of bacterial hyperstructures.Microbiol Mol Biol Rev. 2007 Mar;71(1):230-53. doi: 10.1128/MMBR.00035-06. Microbiol Mol Biol Rev. 2007. PMID: 17347523 Free PMC article. Review.
-
Comparative genomics of the FtsK-HerA superfamily of pumping ATPases: implications for the origins of chromosome segregation, cell division and viral capsid packaging.Nucleic Acids Res. 2004 Oct 5;32(17):5260-79. doi: 10.1093/nar/gkh828. Print 2004. Nucleic Acids Res. 2004. PMID: 15466593 Free PMC article.
-
The bacterial cell cycle, chromosome inheritance and cell growth.Nat Rev Microbiol. 2019 Aug;17(8):467-478. doi: 10.1038/s41579-019-0212-7. Nat Rev Microbiol. 2019. PMID: 31164753 Review.
-
Viral and cellular SOS-regulated motor proteins: dsDNA translocation mechanisms with divergent functions.Cell Biosci. 2014 Jun 25;4:31. doi: 10.1186/2045-3701-4-31. eCollection 2014. Cell Biosci. 2014. PMID: 24995125 Free PMC article. Review.
-
Large chromosomal rearrangements during a long-term evolution experiment with Escherichia coli.mBio. 2014 Sep 9;5(5):e01377-14. doi: 10.1128/mBio.01377-14. mBio. 2014. PMID: 25205090 Free PMC article.
References
-
- Abremski K., Hoess,R. and Sternberg,N. (1983) Studies on the properties of P1 site-specific recombination: evidence for topologically unlinked products following recombination. Cell, 32, 1301–1311. - PubMed
-
- Abremski K., Frommer,B. and Hoess,R.H. (1986) Linking-number changes in the DNA substrate during Cre-mediated loxP site-specific recombination. J. Mol. Biol., 192, 17–26. - PubMed
-
- Adams D.E., Shekhtman,E.M., Zechiedrich,E.L., Schmid,M.B. and Cozzarelli,N.R. (1992) The role of topoisomerase IV in partitioning bacterial replicons and the structure of catenated intermediates in DNA replication. Cell, 71, 277–288. - PubMed
-
- Aussel L., Barre,F.X., Aroyo,M., Stasiak,A., Stasiak,A.Z. and Sherratt,D. (2002) FtsK is a DNA motor protein that activates chromosome dimer resolution by switching the catalytic state of the XerC and XerD recombinases. Cell, 108, 195–205. - PubMed
Publication types
MeSH terms
Substances
LinkOut - more resources
Full Text Sources
Other Literature Sources