GLUT4 is retained by an intracellular cycle of vesicle formation and fusion with endosomes
- PMID: 14595108
- PMCID: PMC329400
- DOI: 10.1091/mbc.e03-07-0517
GLUT4 is retained by an intracellular cycle of vesicle formation and fusion with endosomes
Abstract
The intracellularly stored GLUT4 glucose transporter is rapidly translocated to the cell surface upon insulin stimulation. Regulation of GLUT4 distribution is key for the maintenance of whole body glucose homeostasis. We find that GLUT4 is excluded from the plasma membrane of adipocytes by a dynamic retention/retrieval mechanism. Our kinetic studies indicate that GLUT4-containing vesicles continually bud and fuse with endosomes in the absence of insulin and that these GLUT4 vesicles are 5 times as likely to fuse with an endosome as with the plasma membrane. We hypothesize that this intracellular cycle of vesicle budding and fusion is an element of the active mechanism by which GLUT4 is retained. The GLUT4 trafficking pathway does not extensively overlap with that of furin, indicating that the trans-Golgi network, a compartment in which furin accumulates, is not a significant storage reservoir of GLUT4. An intact microtubule cytoskeleton is required for insulin-stimulated recruitment to the cell surface, although it is not required for the basal budding/fusion cycle. Nocodazole disruption of the microtubule cytoskeleton reduces the insulin-stimulated exocytosis of GLUT4, accounting for the reduced insulin-stimulated translocation of GLUT4 to the cell surface.
Figures
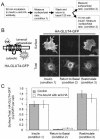
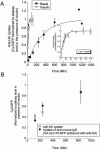
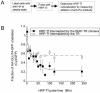
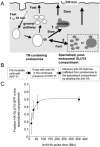
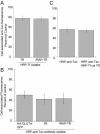
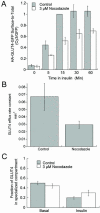
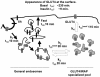
Similar articles
-
Role for the microtubule cytoskeleton in GLUT4 vesicle trafficking and in the regulation of insulin-stimulated glucose uptake.Biochem J. 2000 Dec 1;352 Pt 2(Pt 2):267-76. Biochem J. 2000. PMID: 11085918 Free PMC article.
-
Insulin stimulates the halting, tethering, and fusion of mobile GLUT4 vesicles in rat adipose cells.J Cell Biol. 2005 May 9;169(3):481-9. doi: 10.1083/jcb.200412069. Epub 2005 May 2. J Cell Biol. 2005. PMID: 15866888 Free PMC article.
-
Insulin recruits GLUT4 from specialized VAMP2-carrying vesicles as well as from the dynamic endosomal/trans-Golgi network in rat adipocytes.Mol Biol Cell. 2000 Dec;11(12):4079-91. doi: 10.1091/mbc.11.12.4079. Mol Biol Cell. 2000. PMID: 11102509 Free PMC article.
-
Targeting motifs in GLUT4 (review).Mol Membr Biol. 2001 Oct-Dec;18(4):257-64. doi: 10.1080/09687680110090780. Mol Membr Biol. 2001. PMID: 11780754 Review.
-
Subcellular localization and trafficking of the GLUT4 glucose transporter isoform in insulin-responsive cells.Bioessays. 1994 Oct;16(10):753-9. doi: 10.1002/bies.950161010. Bioessays. 1994. PMID: 7980479 Review.
Cited by
-
Golgin-160 is required for the Golgi membrane sorting of the insulin-responsive glucose transporter GLUT4 in adipocytes.Mol Biol Cell. 2006 Dec;17(12):5346-55. doi: 10.1091/mbc.e06-05-0386. Epub 2006 Oct 18. Mol Biol Cell. 2006. PMID: 17050738 Free PMC article.
-
GLUT4 is sorted to vesicles whose accumulation beneath and insertion into the plasma membrane are differentially regulated by insulin and selectively affected by insulin resistance.Mol Biol Cell. 2010 Apr 15;21(8):1375-86. doi: 10.1091/mbc.e09-08-0751. Epub 2010 Feb 24. Mol Biol Cell. 2010. PMID: 20181829 Free PMC article.
-
Insulin and hypertonicity recruit GLUT4 to the plasma membrane of muscle cells by using N-ethylmaleimide-sensitive factor-dependent SNARE mechanisms but different v-SNAREs: role of TI-VAMP.Mol Biol Cell. 2004 Dec;15(12):5565-73. doi: 10.1091/mbc.e04-03-0266. Epub 2004 Oct 6. Mol Biol Cell. 2004. PMID: 15469990 Free PMC article.
-
Muscling in on GLUT4 kinetics.Commun Integr Biol. 2010 May;3(3):260-2. doi: 10.4161/cib.3.3.11457. Commun Integr Biol. 2010. PMID: 20714409 Free PMC article.
-
High basal cell surface levels of fish GLUT4 are related to reduced sensitivity of insulin-induced translocation toward GGA and AS160 inhibition in adipocytes.Am J Physiol Endocrinol Metab. 2010 Feb;298(2):E329-36. doi: 10.1152/ajpendo.00547.2009. Am J Physiol Endocrinol Metab. 2010. PMID: 20075431 Free PMC article.
References
-
- Barrett, P., Bell, B., Cobelli, C., Golde, H., Schumitzky, A., Vicini, P., and Foster, D. (1998). SAAM II: simulation, analysis, and modeling software for tracer and pharmacokinetic studies. Metabolism 47, 484-492. - PubMed
-
- Bosshart, H., Humphrey, J., Deignan, E., Davidson, J., Drazba, J., Yuan, L., Oorschot, V., Peters, P.J., and Bonifacino, J.S. (1994). The cytoplasmic domain mediates localization of furin to the trans-Golgi network en route to the endosomal/lysosomal system. J. Cell Biol. 126, 1157-1172. - PMC - PubMed
-
- Brown, D. (2003). The ins and outs of aquaporin-2 trafficking. Am. J. Physiol. Renal. Physiol. 284, F893-F901. - PubMed
-
- Bryant, N., Govers, R., and James, D. (2002). Regulated transport of the glucose transporter GLUT4. Nat. Rev. Mol. Cell. Biol. 3, p267-277. - PubMed
-
- Cobelli, C., and Foster, D. (1998). Compartmental models: theory and practice using the SAAM II software system. Adv. Exp. Med. Biol. 445, 79-101. - PubMed
Publication types
MeSH terms
Substances
Grants and funding
LinkOut - more resources
Full Text Sources
Other Literature Sources