A new retroelement constituted by a natural alternatively spliced RNA of murine replication-competent retroviruses
- PMID: 12970198
- PMCID: PMC212718
- DOI: 10.1093/emboj/cdg450
A new retroelement constituted by a natural alternatively spliced RNA of murine replication-competent retroviruses
Erratum in
- EMBO J. 2003 Nov 3;22(21):5962
Abstract
Replication of simple retroviruses depends on the recruitment of a single large primary transcript toward splicing, transport/packaging and translation regulations. In this respect, we studied the novel SD' 4.4 kb RNA of murine leukemia retroviruses (MLV) which results from alternative splicing of the primary transcript. We showed that SD' RNA was required for optimal replication since expression of a pre-spliced SD' RNA trans-complemented the impaired infectivity of a SD'-defective mutant. We monitored the fate of this novel transcript throughout early and late events of the viral life cycle. SD' RNA was specifically incorporated into virions demonstrating that the unspliced RNA was not the unique viral RNA present in virions. Furthermore, SD' RNA was reverse transcribed and its DNA copy integrated into the host genome, thus constituting a new splice donor-associated retroelement (SDARE) in infected cells. Finally, we showed that SD' mRNA encoded a 50 kDa polyprotein, and to a lower extent an additional 60 kDa polyprotein, which harbored Gag and integrase domains.
Figures
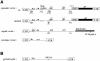
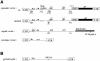
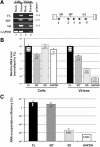
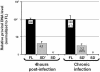

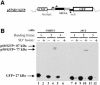
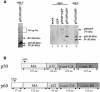
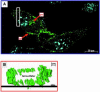
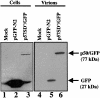
Similar articles
-
Characterization of a natural heterodimer between MLV genomic RNA and the SD' retroelement generated by alternative splicing.RNA. 2007 Dec;13(12):2266-76. doi: 10.1261/rna.713807. Epub 2007 Oct 10. RNA. 2007. PMID: 17928575 Free PMC article.
-
Murine leukemia virus RNA dimerization is coupled to transcription and splicing processes.Retrovirology. 2010 Aug 5;7:64. doi: 10.1186/1742-4690-7-64. Retrovirology. 2010. PMID: 20687923 Free PMC article.
-
Activation of c-myb by 5' retrovirus promoter insertion in myeloid neoplasms is dependent upon an intact alternative splice donor site (SD') in gag.Virology. 2004 Dec 20;330(2):398-407. doi: 10.1016/j.virol.2004.09.038. Virology. 2004. PMID: 15567434
-
Insights into the nuclear export of murine leukemia virus intron-containing RNA.RNA Biol. 2015;12(9):942-9. doi: 10.1080/15476286.2015.1065375. RNA Biol. 2015. PMID: 26158194 Free PMC article. Review.
-
Chapter 1. Regulation of HIV-1 alternative RNA splicing and its role in virus replication.Adv Virus Res. 2009;74:1-40. doi: 10.1016/S0065-3527(09)74001-1. Adv Virus Res. 2009. PMID: 19698894 Review.
Cited by
-
Impairment of alternative splice sites defining a novel gammaretroviral exon within gag modifies the oncogenic properties of Akv murine leukemia virus.Retrovirology. 2007 Jul 6;4:46. doi: 10.1186/1742-4690-4-46. Retrovirology. 2007. PMID: 17617899 Free PMC article.
-
Pseudodiploid genome organization AIDS full-length human immunodeficiency virus type 1 DNA synthesis.J Virol. 2008 Mar;82(5):2376-84. doi: 10.1128/JVI.02100-07. Epub 2007 Dec 19. J Virol. 2008. PMID: 18094172 Free PMC article.
-
Characterization of a natural heterodimer between MLV genomic RNA and the SD' retroelement generated by alternative splicing.RNA. 2007 Dec;13(12):2266-76. doi: 10.1261/rna.713807. Epub 2007 Oct 10. RNA. 2007. PMID: 17928575 Free PMC article.
-
MoMuLV and HIV-1 nucleocapsid proteins have a common role in genomic RNA packaging but different in late reverse transcription.PLoS One. 2012;7(12):e51534. doi: 10.1371/journal.pone.0051534. Epub 2012 Dec 7. PLoS One. 2012. PMID: 23236513 Free PMC article.
-
From Cells to Virus Particles: Quantitative Methods to Monitor RNA Packaging.Viruses. 2016 Aug 22;8(8):239. doi: 10.3390/v8080239. Viruses. 2016. PMID: 27556480 Free PMC article. Review.
References
-
- Alin K. and Goff,S.P. (1996a) Amino acid substitutions in the CA protein of Moloney murine leukemia virus that block early events in infection. Virology, 222, 339–351. - PubMed
-
- Alin K. and Goff,S.P. (1996b) Mutational analysis of interactions between the Gag precursor proteins of murine leukemia viruses. Virology, 216, 418–424. - PubMed
Publication types
MeSH terms
Substances
LinkOut - more resources
Full Text Sources