Longevity regulation in Saccharomyces cerevisiae: linking metabolism, genome stability, and heterochromatin
- PMID: 12966141
- PMCID: PMC193872
- DOI: 10.1128/MMBR.67.3.376-399.2003
Longevity regulation in Saccharomyces cerevisiae: linking metabolism, genome stability, and heterochromatin
Abstract
When it was first proposed that the budding yeast Saccharomyces cerevisiae might serve as a model for human aging in 1959, the suggestion was met with considerable skepticism. Although yeast had proved a valuable model for understanding basic cellular processes in humans, it was difficult to accept that such a simple unicellular organism could provide information about human aging, one of the most complex of biological phenomena. While it is true that causes of aging are likely to be multifarious, there is a growing realization that all eukaryotes possess surprisingly conserved longevity pathways that govern the pace of aging. This realization has come, in part, from studies of S. cerevisiae, which has emerged as a highly informative and respected model for the study of life span regulation. Genomic instability has been identified as a major cause of aging, and over a dozen longevity genes have now been identified that suppress it. Here we present the key discoveries in the yeast-aging field, regarding both the replicative and chronological measures of life span in this organism. We discuss the implications of these findings not only for mammalian longevity but also for other key aspects of cell biology, including cell survival, the relationship between chromatin structure and genome stability, and the effect of internal and external environments on cellular defense pathways. We focus on the regulation of replicative life span, since recent findings have shed considerable light on the mechanisms controlling this process. We also present the specific methods used to study aging and longevity regulation in S. cerevisiae.
Figures
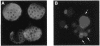
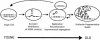
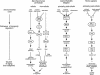
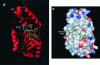
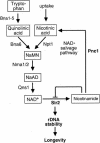
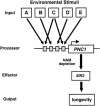
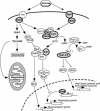
Similar articles
-
Life-span extension in yeast.Science. 2006 Apr 14;312(5771):195-7; author reply 195-7. doi: 10.1126/science.312.5771.195d. Science. 2006. PMID: 16614198 No abstract available.
-
Cell biology. Twists in the tale of the aging yeast.Science. 2005 Nov 18;310(5751):1124-5. doi: 10.1126/science.1121310. Science. 2005. PMID: 16293743 No abstract available.
-
Sir2 blocks extreme life-span extension.Cell. 2005 Nov 18;123(4):655-67. doi: 10.1016/j.cell.2005.08.042. Cell. 2005. PMID: 16286010
-
Sir2 and calorie restriction in yeast: a skeptical perspective.Ageing Res Rev. 2007 Aug;6(2):128-40. doi: 10.1016/j.arr.2007.04.001. Epub 2007 Apr 19. Ageing Res Rev. 2007. PMID: 17512264 Review.
-
[The molecular mechanism of aging and longevity and the function of Sir2 proteins].Nihon Ronen Igakkai Zasshi. 2001 Nov;38(6):735-9. Nihon Ronen Igakkai Zasshi. 2001. PMID: 11774709 Review. Japanese. No abstract available.
Cited by
-
Protein aggregation activates erratic stress response in dietary restricted yeast cells.Sci Rep. 2016 Sep 16;6:33433. doi: 10.1038/srep33433. Sci Rep. 2016. PMID: 27633120 Free PMC article.
-
Progress in metabolic engineering of Saccharomyces cerevisiae.Microbiol Mol Biol Rev. 2008 Sep;72(3):379-412. doi: 10.1128/MMBR.00025-07. Microbiol Mol Biol Rev. 2008. PMID: 18772282 Free PMC article. Review.
-
Respiratory and TCA cycle activities affect S. cerevisiae lifespan, response to caloric restriction and mtDNA stability.J Bioenerg Biomembr. 2011 Oct;43(5):483-91. doi: 10.1007/s10863-011-9377-0. Epub 2011 Jul 21. J Bioenerg Biomembr. 2011. PMID: 21833600
-
A network biology approach to aging in yeast.Proc Natl Acad Sci U S A. 2009 Jan 27;106(4):1145-50. doi: 10.1073/pnas.0812551106. Epub 2009 Jan 21. Proc Natl Acad Sci U S A. 2009. PMID: 19164565 Free PMC article.
-
Age-dependent changes in the sphingolipid composition of mouse CD4+ T cell membranes and immune synapses implicate glucosylceramides in age-related T cell dysfunction.PLoS One. 2012;7(10):e47650. doi: 10.1371/journal.pone.0047650. Epub 2012 Oct 26. PLoS One. 2012. PMID: 23110086 Free PMC article.
References
-
- Aguilaniu, H., L. Gustafsson, M. Rigoulet, and T. Nystrom. 2003. Asymmetric inheritance of oxidatively damaged proteins during cytokinesis. Science 299:1751-1753. - PubMed
-
- Anderson, R. M., K. J. Bitterman, J. G. Wood, O. Medvedik, H. Cohen, S. S. Lin, J. K. Manchester, J. I. Gordon, and D. A. Sinclair. 2002. Manipulation of a nuclear NAD+ salvage pathway delays aging without altering steady-state NAD+ levels. J. Biol. Chem. 277:18881-18890. - PubMed
-
- Reference deleted.
Publication types
MeSH terms
Substances
Grants and funding
- P01 AG027916-030003/AG/NIA NIH HHS/United States
- P01 AG027916-020003/AG/NIA NIH HHS/United States
- P01 AG027916-010003/AG/NIA NIH HHS/United States
- P01 AG027916-04S20003/AG/NIA NIH HHS/United States
- R01 AG019719-06A1/AG/NIA NIH HHS/United States
- R01 AG028730-03/AG/NIA NIH HHS/United States
- P01 AG027916/AG/NIA NIH HHS/United States
- P01 AG027916-04S10003/AG/NIA NIH HHS/United States
- R01 AG028730/AG/NIA NIH HHS/United States
- R01 AG019719-07/AG/NIA NIH HHS/United States
- R01 AG028730-02/AG/NIA NIH HHS/United States
- R01 AG028730-01A1/AG/NIA NIH HHS/United States
- P01 AG027916-040003/AG/NIA NIH HHS/United States
- R01 AG019719/AG/NIA NIH HHS/United States
LinkOut - more resources
Full Text Sources
Other Literature Sources
Molecular Biology Databases
Miscellaneous