Involvement of the secretory pathway and the cytoskeleton in intracellular targeting and tubule assembly of Grapevine fanleaf virus movement protein in tobacco BY-2 cells
- PMID: 12953111
- PMCID: PMC181331
- DOI: 10.1105/tpc.013896
Involvement of the secretory pathway and the cytoskeleton in intracellular targeting and tubule assembly of Grapevine fanleaf virus movement protein in tobacco BY-2 cells
Abstract
Grapevine fanleaf virus (GFLV) is one of a large class of plant viruses whose cell-to-cell transport involves the passage of virions through tubules composed of virus-encoded movement protein (MP). The tubules are embedded within modified plasmodesmata, but the mechanism of targeting of MP to these sites is unknown. To study intracellular GFLV MP trafficking, a green fluorescent protein-MP fusion (GFP:MP) was expressed in transgenic tobacco BY-2 suspension cells under the control of an inducible promoter. We show that GFP:MP is targeted preferentially to calreticulin-labeled foci within the youngest cross walls, where it assembles into tubules. During cell division, GFP:MP colocalizes in the cell plate with KNOLLE, a cytokinesis-specific syntaxin, and both proteins are linked physically, as shown by coimmunoprecipitation of the two proteins from the same microsomal fraction. In addition, treatment with various drugs has revealed that a functional secretory pathway, but not the cytoskeleton, is required for tubule formation. However, correct GFP:MP targeting to calreticulin-labeled foci seems to be cytoskeleton dependent. Finally, biochemical analyses have revealed that at least a fraction of the MP behaves as an intrinsic membrane protein. These findings support a model in which GFP:MP would be transported to specific sites via Golgi-derived vesicles along two different pathways: a microtubule-dependent pathway in normal cells and a microfilament-dependent default pathway when microtubules are depolymerized.
Figures
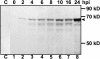
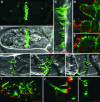
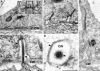
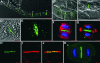
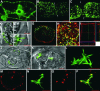
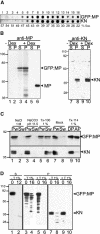
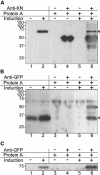
Similar articles
-
The cytoskeleton and the secretory pathway are not involved in targeting the cowpea mosaic virus movement protein to the cell periphery.Virology. 2002 May 25;297(1):48-56. doi: 10.1006/viro.2002.1424. Virology. 2002. PMID: 12083835
-
Interaction of tobamovirus movement proteins with the plant cytoskeleton.Science. 1995 Dec 22;270(5244):1983-5. doi: 10.1126/science.270.5244.1983. Science. 1995. PMID: 8533089
-
Inhibition of tobacco mosaic virus movement by expression of an actin-binding protein.Plant Physiol. 2009 Apr;149(4):1810-23. doi: 10.1104/pp.108.133827. Epub 2009 Feb 13. Plant Physiol. 2009. PMID: 19218363 Free PMC article.
-
Cytoplasmic illuminations: in planta targeting of fluorescent proteins to cellular organelles.Protoplasma. 2001;215(1-4):77-88. doi: 10.1007/BF01280305. Protoplasma. 2001. PMID: 11732067 Review.
-
Protein-protein interactions in the secretory pathway, a growing demand for experimental approaches in vivo.Plant Mol Biol. 2002 Dec;50(6):887-902. doi: 10.1023/a:1021266320877. Plant Mol Biol. 2002. PMID: 12516860 Review.
Cited by
-
Interference of Brefeldin A in viral movement protein tubules assembly.Plant Signal Behav. 2014;9(7):e29121. doi: 10.4161/psb.29121. Plant Signal Behav. 2014. PMID: 25763491 Free PMC article.
-
Elucidating the Subcellular Localization of GLRaV-3 Proteins Encoded by the Unique Gene Block in N. benthamiana Suggests Implications on Plant Host Suppression.Biomolecules. 2024 Aug 9;14(8):977. doi: 10.3390/biom14080977. Biomolecules. 2024. PMID: 39199365 Free PMC article.
-
AtCCMH, an essential component of the c-type cytochrome maturation pathway in Arabidopsis mitochondria, interacts with apocytochrome c.Proc Natl Acad Sci U S A. 2005 Nov 1;102(44):16113-8. doi: 10.1073/pnas.0503473102. Epub 2005 Oct 18. Proc Natl Acad Sci U S A. 2005. PMID: 16236729 Free PMC article.
-
Inspirations on Virus Replication and Cell-to-Cell Movement from Studies Examining the Cytopathology Induced by Lettuce infectious yellows virus in Plant Cells.Front Plant Sci. 2017 Sep 27;8:1672. doi: 10.3389/fpls.2017.01672. eCollection 2017. Front Plant Sci. 2017. PMID: 29021801 Free PMC article. Review.
-
Phloem Exudate Protein Profiles during Drought and Recovery Reveal Abiotic Stress Responses in Tomato Vasculature.Int J Mol Sci. 2020 Jun 23;21(12):4461. doi: 10.3390/ijms21124461. Int J Mol Sci. 2020. PMID: 32586033 Free PMC article.
References
-
- Akashi, T., Izumi, K., Nagano, E., Enomoto, M., Mizuno, K., and Shibaoka, H. (1988). Effects of propyzamide on tobacco cell microtubules in vivo and in vitro. Plant Cell Physiol. 29, 1053–1062.
-
- Aoyama, T., and Chua, N. (1997). A glucocorticoid-mediated transcriptional induction system in transgenic plants. Plant J. 11, 605–612. - PubMed
-
- Baluška, F., Samaj, J., Napier, R., and Volkmann, D. (1999). Maize calreticulin localizes preferentially to plasmodesmata in root apex. Plant J. 19, 481–488. - PubMed
-
- Bertens, P., Wellink, J., Goldbach, R., and van Kammen, A. (2000). Mutational analysis of the cowpea mosaic virus movement protein. Virology 267, 199–208. - PubMed
Publication types
MeSH terms
Substances
LinkOut - more resources
Full Text Sources
Research Materials