Tumors acquire inhibitor of apoptosis protein (IAP)-mediated apoptosis resistance through altered specificity of cytosolic proteolysis
- PMID: 12810691
- PMCID: PMC2193958
- DOI: 10.1084/jem.20020801
Tumors acquire inhibitor of apoptosis protein (IAP)-mediated apoptosis resistance through altered specificity of cytosolic proteolysis
Abstract
Many tumors overexpress members of the inhibitor of apoptosis protein (IAP) family. IAPs contribute to tumor cell apoptosis resistance by the inhibition of caspases, and are degraded by the proteasome to allow further progression of apoptosis. Here we show that tumor cells can alter the specificity of cytosolic proteolysis in order to acquire apoptosis resistance, which promotes formation of rapidly growing tumors. Survival of tumor cells with low proteasomal activity can occur in the presence of high expression of Tri-peptidyl-peptidase II (TPP II), a large subtilisin-like peptidase that complements proteasomal activity. We find that this state leaves tumor cells unable of effectively degrading IAPs, and that cells in this state form rapidly growing tumors in vivo. We also find, in studies of apoptosis resistant cells derived from large in vivo tumors, that these have acquired an altered peptidase activity, with up-regulation of TPP II activity and decreased proteasomal activity. Importantly, we find that growth of subcutaneous tumors is limited by maintenance of the apoptosis resistant phenotype. The apoptosis resistant phenotype was reversed by increased expression of Smac/DIABLO, an antagonist of IAP molecules. Our data suggest a reversible mechanism in regulation of apoptosis resistance that drives tumor progression in vivo. These data are relevant in relation to the multitude of therapy-resistant clinical tumors that have increased levels of IAP molecules.
Figures
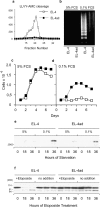
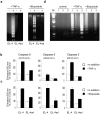
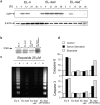
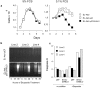
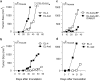
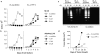
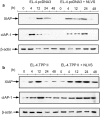
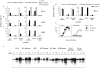
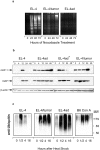
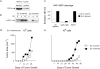
Similar articles
-
c-myc overexpression activates alternative pathways for intracellular proteolysis in lymphoma cells.Nat Cell Biol. 2001 Mar;3(3):283-8. doi: 10.1038/35060076. Nat Cell Biol. 2001. PMID: 11231578
-
Endogenously released Smac is insufficient to mediate cell death of human lung carcinoma in response to etoposide.Exp Cell Res. 2004 Aug 1;298(1):83-95. doi: 10.1016/j.yexcr.2004.04.007. Exp Cell Res. 2004. PMID: 15242764
-
Antitumor effect of the human immunodeficiency virus protease inhibitor ritonavir: induction of tumor-cell apoptosis associated with perturbation of proteasomal proteolysis.Cancer Res. 2002 Dec 1;62(23):6901-8. Cancer Res. 2002. PMID: 12460905
-
Proteasomes get by with lots of help from their friends.Immunity. 2004 Apr;20(4):362-3. doi: 10.1016/s1074-7613(04)00086-x. Immunity. 2004. PMID: 15084265 Review.
-
Molecular regulation of muscle cachexia: it may be more than the proteasome.Biochem Biophys Res Commun. 2002 Jan 11;290(1):1-10. doi: 10.1006/bbrc.2001.5849. Biochem Biophys Res Commun. 2002. PMID: 11779124 Review.
Cited by
-
Early-onset Evans syndrome, immunodeficiency, and premature immunosenescence associated with tripeptidyl-peptidase II deficiency.Blood. 2015 Jan 29;125(5):753-61. doi: 10.1182/blood-2014-08-593202. Epub 2014 Nov 20. Blood. 2015. PMID: 25414442 Free PMC article.
-
The Enigma of Tripeptidyl-Peptidase II: Dual Roles in Housekeeping and Stress.J Oncol. 2010;2010:128478. doi: 10.1155/2010/128478. Epub 2010 Aug 18. J Oncol. 2010. PMID: 20847939 Free PMC article.
-
Molecular architecture and assembly mechanism of Drosophila tripeptidyl peptidase II.Proc Natl Acad Sci U S A. 2005 Jul 19;102(29):10135-40. doi: 10.1073/pnas.0504569102. Epub 2005 Jul 8. Proc Natl Acad Sci U S A. 2005. PMID: 16006508 Free PMC article.
-
Post-proteasomal and proteasome-independent generation of MHC class I ligands.Cell Mol Life Sci. 2011 May;68(9):1553-67. doi: 10.1007/s00018-011-0662-1. Epub 2011 Mar 10. Cell Mol Life Sci. 2011. PMID: 21390545 Free PMC article. Review.
-
Activation of cellular death programs associated with immunosenescence-like phenotype in TPPII knockout mice.Proc Natl Acad Sci U S A. 2008 Apr 1;105(13):5177-82. doi: 10.1073/pnas.0801413105. Epub 2008 Mar 24. Proc Natl Acad Sci U S A. 2008. PMID: 18362329 Free PMC article.
References
-
- Rock, K.L., and A.L. Goldberg. 1999. Degradation of cell proteins and the generation of MHC class I-presented peptides. Annu. Rev. Immunol. 17:739–779. - PubMed
-
- Schwartz, A.L., and A. Ciechanover. 1999. The ubiquitin-proteasome pathway and pathogenesis of human diseases. Annu. Rev. Med. 50:57–74. - PubMed
-
- Voges, D., P. Zwickl, and W. Baumeister. 1999. The 26S proteasome: a molecular machine designed for controlled proteolysis. Annu. Rev. Biochem. 68:1015–1068. - PubMed
-
- Dantuma, N.P., K. Lindsten, R. Glas, M. Jellne, and M.G. Masucci. 2000. Short-lived green fluorescent proteins for quantifying ubiquitin/proteasome-dependent proteolysis in living cells. Nat. Biotechnol. 18:538–543. - PubMed
Publication types
MeSH terms
Substances
LinkOut - more resources
Full Text Sources
Other Literature Sources
Research Materials