Rae1 is an essential mitotic checkpoint regulator that cooperates with Bub3 to prevent chromosome missegregation
- PMID: 12551952
- PMCID: PMC2172680
- DOI: 10.1083/jcb.200211048
Rae1 is an essential mitotic checkpoint regulator that cooperates with Bub3 to prevent chromosome missegregation
Abstract
The WD-repeat proteins Rae1 and Bub3 show extensive sequence homology, indicative of functional similarity. However, previous studies have suggested that Rae1 is involved in the mRNA export pathway and Bub3 in the mitotic checkpoint. To determine the in vivo roles of Rae1 and Bub3 in mammals, we generated knockout mice that have these genes deleted individually or in combination. Here we show that haplo-insufficiency of either Rae1 or Bub3 results in a similar phenotype involving mitotic checkpoint defects and chromosome missegregation. We also show that overexpression of Rae1 can correct for Rae1 haplo-insufficiency and, surprisingly, Bub3 haplo-insufficiency. Rae1-null and Bub3-null mice are embryonic lethal, although cells from these mice did not have a detectable defect in nuclear export of mRNA. Unlike null mice, compound haplo-insufficient Rae1/Bub3 mice are viable. However, cells from these mice exhibit much greater rates of premature sister chromatid separation and chromosome missegregation than single haplo-insufficient cells. Finally, we show that mice with mitotic checkpoint defects are more susceptible to dimethylbenzanthrene-induced tumorigenesis than wild-type mice. Thus, our data demonstrate a novel function for Rae1 and characterize Rae1 and Bub3 as related proteins with essential, overlapping, and cooperating roles in the mitotic checkpoint.
Figures
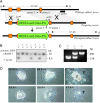
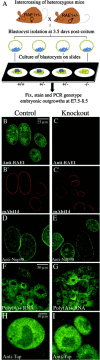
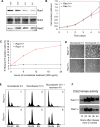
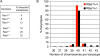
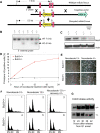
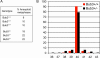
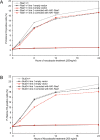
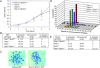
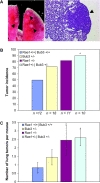
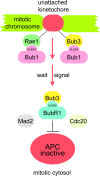
Similar articles
-
Early aging-associated phenotypes in Bub3/Rae1 haploinsufficient mice.J Cell Biol. 2006 Feb 13;172(4):529-40. doi: 10.1083/jcb.200507081. J Cell Biol. 2006. PMID: 16476774 Free PMC article.
-
Aging in check.Sci Aging Knowledge Environ. 2006 Apr 5;2006(7):pe9. doi: 10.1126/sageke.2006.7.pe9. Sci Aging Knowledge Environ. 2006. PMID: 16600919 Review.
-
Differential mitotic checkpoint protein requirements in somatic and germ cells.Biochem Soc Trans. 2006 Aug;34(Pt 4):583-6. doi: 10.1042/BST0340583. Biochem Soc Trans. 2006. PMID: 16856867
-
The Rae1-Nup98 complex prevents aneuploidy by inhibiting securin degradation.Nature. 2005 Dec 15;438(7070):1036-9. doi: 10.1038/nature04221. Nature. 2005. PMID: 16355229
-
Does aneuploidy cause cancer?Curr Opin Cell Biol. 2006 Dec;18(6):658-67. doi: 10.1016/j.ceb.2006.10.002. Epub 2006 Oct 12. Curr Opin Cell Biol. 2006. PMID: 17046232 Review.
Cited by
-
Haploinsufficiency of SGO1 results in deregulated centrosome dynamics, enhanced chromosomal instability and colon tumorigenesis.Cell Cycle. 2012 Feb 1;11(3):479-88. doi: 10.4161/cc.11.3.18994. Epub 2012 Feb 1. Cell Cycle. 2012. PMID: 22262168 Free PMC article.
-
A dominant interfering Bub1 mutant is insufficient to induce or alter thymic tumorigenesis in vivo, even in a sensitized genetic background.Mol Cell Biol. 2005 Sep;25(17):7796-802. doi: 10.1128/MCB.25.17.7796-7802.2005. Mol Cell Biol. 2005. PMID: 16107724 Free PMC article.
-
Rae1 interaction with NuMA is required for bipolar spindle formation.Proc Natl Acad Sci U S A. 2006 Dec 26;103(52):19783-7. doi: 10.1073/pnas.0609582104. Epub 2006 Dec 15. Proc Natl Acad Sci U S A. 2006. PMID: 17172455 Free PMC article.
-
Nup98-homeodomain fusions interact with endogenous Nup98 during interphase and localize to kinetochores and chromosome arms during mitosis.Mol Biol Cell. 2010 May 1;21(9):1585-96. doi: 10.1091/mbc.e09-07-0561. Epub 2010 Mar 17. Mol Biol Cell. 2010. PMID: 20237156 Free PMC article.
-
Inactivating the spindle checkpoint kinase Bub1 during embryonic development results in a global shutdown of proliferation.BMC Res Notes. 2009 Sep 23;2:190. doi: 10.1186/1756-0500-2-190. BMC Res Notes. 2009. PMID: 19772675 Free PMC article.
References
-
- Bachi, A., I.C. Braun, J.P. Rodrigues, N. Pante, K. Ribbeck, C. Von Kobbe, U. Kutay, M. Wilm, D. Gorlich, M. Carmo-Fonseca, and E. Izaurralde. 2000. The C-terminal domain of TAP interacts with the nuclear pore complex and promotes export of specific CTE-bearing RNA substrates. RNA. 6:136–158. - PMC - PubMed
Publication types
MeSH terms
Substances
Grants and funding
LinkOut - more resources
Full Text Sources
Other Literature Sources
Molecular Biology Databases
Miscellaneous