Ribosylnicotinamide kinase domain of NadR protein: identification and implications in NAD biosynthesis
- PMID: 12446641
- PMCID: PMC135457
- DOI: 10.1128/JB.184.24.6906-6917.2002
Ribosylnicotinamide kinase domain of NadR protein: identification and implications in NAD biosynthesis
Erratum in
- J Bacteriol 2003 Jan;185(2):698
Abstract
NAD is an indispensable redox cofactor in all organisms. Most of the genes required for NAD biosynthesis in various species are known. Ribosylnicotinamide kinase (RNK) was among the few unknown (missing) genes involved with NAD salvage and recycling pathways. Using a comparative genome analysis involving reconstruction of NAD metabolism from genomic data, we predicted and experimentally verified that bacterial RNK is encoded within the 3' region of the nadR gene. Based on these results and previous data, the full-size multifunctional NadR protein (as in Escherichia coli) is composed of (i) an N-terminal DNA-binding domain involved in the transcriptional regulation of NAD biosynthesis, (ii) a central nicotinamide mononucleotide adenylyltransferase (NMNAT) domain, and (iii) a C-terminal RNK domain. The RNK and NMNAT enzymatic activities of recombinant NadR proteins from Salmonella enterica serovar Typhimurium and Haemophilus influenzae were quantitatively characterized. We propose a model for the complete salvage pathway from exogenous N-ribosylnicotinamide to NAD which involves the concerted action of the PnuC transporter and NRK, followed by the NMNAT activity of the NadR protein. Both the pnuC and nadR genes were proven to be essential for the growth and survival of H. influenzae, thus implicating them as potential narrow-spectrum drug targets.
Figures
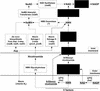
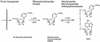
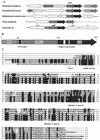
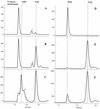
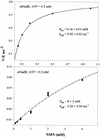
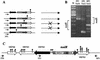
Similar articles
-
Crystal structure of Haemophilus influenzae NadR protein. A bifunctional enzyme endowed with NMN adenyltransferase and ribosylnicotinimide kinase activities.J Biol Chem. 2002 Sep 6;277(36):33291-9. doi: 10.1074/jbc.M204368200. Epub 2002 Jun 14. J Biol Chem. 2002. PMID: 12068016
-
Regulation of NAD metabolism in Salmonella typhimurium: molecular sequence analysis of the bifunctional nadR regulator and the nadA-pnuC operon.J Bacteriol. 1990 Aug;172(8):4187-96. doi: 10.1128/jb.172.8.4187-4196.1990. J Bacteriol. 1990. PMID: 2198247 Free PMC article.
-
Regulation of NAD synthesis by the trifunctional NadR protein of Salmonella enterica.J Bacteriol. 2005 Apr;187(8):2774-82. doi: 10.1128/JB.187.8.2774-2782.2005. J Bacteriol. 2005. PMID: 15805524 Free PMC article.
-
Comparative genomics of NAD(P) biosynthesis and novel antibiotic drug targets.J Cell Physiol. 2011 Feb;226(2):331-40. doi: 10.1002/jcp.22419. J Cell Physiol. 2011. PMID: 20857400 Review.
-
NAD(P) biosynthesis enzymes as potential targets for selective drug design.Curr Med Chem. 2009;16(11):1372-90. doi: 10.2174/092986709787846505. Curr Med Chem. 2009. PMID: 19355893 Review.
Cited by
-
Quinolinate salvage and insights for targeting NAD biosynthesis in group A streptococci.J Bacteriol. 2013 Feb;195(4):726-32. doi: 10.1128/JB.02002-12. Epub 2012 Nov 30. J Bacteriol. 2013. PMID: 23204464 Free PMC article.
-
Poly(ADP-Ribose) Polymerases in Host-Pathogen Interactions, Inflammation, and Immunity.Microbiol Mol Biol Rev. 2018 Dec 19;83(1):e00038-18. doi: 10.1128/MMBR.00038-18. Print 2019 Mar. Microbiol Mol Biol Rev. 2018. PMID: 30567936 Free PMC article. Review.
-
The transporter-opsin-G protein-coupled receptor (TOG) superfamily.FEBS J. 2013 Nov;280(22):5780-800. doi: 10.1111/febs.12499. Epub 2013 Sep 23. FEBS J. 2013. PMID: 23981446 Free PMC article.
-
Structural analysis and insight into effector binding of the niacin-responsive repressor NiaR from Bacillus halodurans.Sci Rep. 2020 Dec 3;10(1):21039. doi: 10.1038/s41598-020-78148-x. Sci Rep. 2020. PMID: 33273654 Free PMC article.
-
Assimilation of nicotinamide mononucleotide requires periplasmic AphA phosphatase in Salmonella enterica.J Bacteriol. 2005 Jul;187(13):4521-30. doi: 10.1128/JB.187.13.4521-4530.2005. J Bacteriol. 2005. PMID: 15968063 Free PMC article.
References
-
- Ausubel, F. M. 1999. Short protocols in molecular biology: a compendium of methods from Current protocols in molecular biology, 4th ed. Wiley, New York, N.Y.
-
- Balducci, E., M. Emanuelli, N. Raffaelli, S. Ruggieri, A. Amici, G. Magni, G. Orsomando, V. Polzonetti, and P. Natalini. 1995. Assay methods for nicotinamide mononucleotide adenylyltransferase of wide applicability. Anal. Biochem. 228:64-68. - PubMed
-
- Barcak, G. J., M. S. Chandler, R. J. Redfield, and J. F. Tomb. 1991. Genetic systems in Haemophilus influenzae. Methods Enzymol. 204:321-342. - PubMed
MeSH terms
Substances
LinkOut - more resources
Full Text Sources
Other Literature Sources
Molecular Biology Databases