The membrane-proximal domain of vesicular stomatitis virus G protein functions as a membrane fusion potentiator and can induce hemifusion
- PMID: 12414970
- PMCID: PMC136858
- DOI: 10.1128/jvi.76.23.12300-12311.2002
The membrane-proximal domain of vesicular stomatitis virus G protein functions as a membrane fusion potentiator and can induce hemifusion
Abstract
Recently we showed that the membrane-proximal stem region of the vesicular stomatitis virus (VSV) G protein ectodomain (G stem [GS]), together with the transmembrane and cytoplasmic domains, was sufficient to mediate efficient VSV budding (C. S. Robison and M. A. Whitt, J. Virol. 74:2239-2246, 2000). Here, we show that GS can also potentiate the membrane fusion activity of heterologous viral fusion proteins when GS is coexpressed with those proteins. For some fusion proteins, there was as much as a 40-fold increase in syncytium formation when GS was coexpressed compared to that seen when the fusion protein was expressed alone. Fusion potentiation by GS was not protein specific, since it occurred with both pH-dependent as well as pH-independent fusion proteins. Using a recombinant vesicular stomatitis virus encoding GS that contained an N-terminal hemagglutinin (HA) tag (GS(HA) virus), we found that the GS(HA) virus bound to cells as well as the wild-type virus did at pH 7.0; however, the GS(HA) virus was noninfectious. Analysis of cells expressing GS(HA) in a three-color membrane fusion assay revealed that GS(HA) could induce lipid mixing but not cytoplasmic mixing, indicating that GS can induce hemifusion. Treatment of GS(HA) virus-bound cells with the membrane-destabilizing drug chlorpromazine rescued the hemifusion block and allowed entry and subsequent replication of GS(HA) virus, demonstrating that GS-mediated hemifusion was a functional intermediate in the membrane fusion pathway. Using a series of truncation mutants, we also determined that only 14 residues of GS, together with the VSV G transmembrane and cytoplasmic tail, were sufficient for fusion potentiation. To our knowledge, this is the first report which shows that a small domain of one viral glycoprotein can promote the fusion activity of other, unrelated viral glycoproteins.
Figures
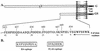
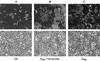
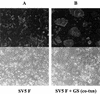
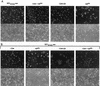
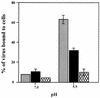
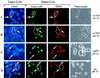
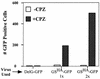
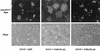
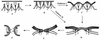
Similar articles
-
The membrane-proximal region of vesicular stomatitis virus glycoprotein G ectodomain is critical for fusion and virus infectivity.J Virol. 2003 Dec;77(23):12807-18. doi: 10.1128/jvi.77.23.12807-12818.2003. J Virol. 2003. PMID: 14610202 Free PMC article.
-
A recombinant vesicular stomatitis virus bearing a lethal mutation in the glycoprotein gene uncovers a second site suppressor that restores fusion.J Virol. 2011 Aug;85(16):8105-15. doi: 10.1128/JVI.00735-11. Epub 2011 Jun 15. J Virol. 2011. PMID: 21680501 Free PMC article.
-
Important role for the transmembrane domain of severe acute respiratory syndrome coronavirus spike protein during entry.J Virol. 2006 Feb;80(3):1302-10. doi: 10.1128/JVI.80.3.1302-1310.2006. J Virol. 2006. PMID: 16415007 Free PMC article.
-
Structures of vesicular stomatitis virus glycoprotein: membrane fusion revisited.Cell Mol Life Sci. 2008 Jun;65(11):1716-28. doi: 10.1007/s00018-008-7534-3. Cell Mol Life Sci. 2008. PMID: 18345480 Free PMC article. Review.
-
Viruses as model systems in cell biology.Methods Cell Biol. 1994;43 Pt A:3-42. doi: 10.1016/s0091-679x(08)60596-8. Methods Cell Biol. 1994. PMID: 7823868 Free PMC article. Review.
Cited by
-
Phosphatidylserine orchestrates Myomerger membrane insertions to drive myoblast fusion.Proc Natl Acad Sci U S A. 2022 Sep 20;119(38):e2202490119. doi: 10.1073/pnas.2202490119. Epub 2022 Sep 12. Proc Natl Acad Sci U S A. 2022. PMID: 36095199 Free PMC article.
-
Entry of rhabdoviruses into animal cells.Adv Exp Med Biol. 2013;790:167-77. doi: 10.1007/978-1-4614-7651-1_9. Adv Exp Med Biol. 2013. PMID: 23884591 Free PMC article. Review.
-
Structural and functional roles of HIV-1 gp41 pretransmembrane sequence segmentation.Biophys J. 2003 Dec;85(6):3769-80. doi: 10.1016/S0006-3495(03)74792-4. Biophys J. 2003. PMID: 14645067 Free PMC article.
-
Structures and mechanisms of viral membrane fusion proteins: multiple variations on a common theme.Crit Rev Biochem Mol Biol. 2008 May-Jun;43(3):189-219. doi: 10.1080/10409230802058320. Crit Rev Biochem Mol Biol. 2008. PMID: 18568847 Free PMC article. Review.
-
Class III viral membrane fusion proteins.Curr Opin Struct Biol. 2009 Apr;19(2):189-96. doi: 10.1016/j.sbi.2009.02.012. Epub 2009 Apr 6. Curr Opin Struct Biol. 2009. PMID: 19356922 Free PMC article. Review.
References
-
- Berger, E. A., P. M. Murphy, and J. M. Farber. 1999. Chemokine receptors as HIV-1 coreceptors: roles in viral entry, tropism, and disease. Annu. Rev. Immunol. 17:657-700. - PubMed
-
- Blumenthal, R., A. Bali-Puri, A. Walter, D. Covell, and O. Eidelman. 1987. pH-dependent fusion of vesicular stomatitis virus with Vero cells. Measurement by dequenching of octadecyl rhodamine fluorescence. J. Biol. Chem. 262:13614-13619. - PubMed
-
- Bricker, B. J., R. M. Snyder, J. W. Fox, W. A. Volk, and R. R. Wagner. 1987. Monoclonal antibodies to the glycoprotein of vesicular stomatitis virus (New Jersey serotype): a method for preliminary mapping of epitopes. Virology 161:533-540. - PubMed
-
- Broder, C. C., D. S. Dimitrov, R. Blumenthal, and E. A. Berger. 1993. The block to HIV-1 envelope glycoprotein-mediated membrane fusion in animal cells expressing human CD4 can be overcome by a human cell component(s). Virology 193:483-491. - PubMed
Publication types
MeSH terms
Substances
Grants and funding
LinkOut - more resources
Full Text Sources
Other Literature Sources