Keren, a new ligand of the Drosophila epidermal growth factor receptor, undergoes two modes of cleavage
- PMID: 12169631
- PMCID: PMC126175
- DOI: 10.1093/emboj/cdf439
Keren, a new ligand of the Drosophila epidermal growth factor receptor, undergoes two modes of cleavage
Abstract
Spitz (Spi) is the most prominent ligand of the Drosophila EGF receptor (DER). It is produced as an inactive membrane precursor which is retained in the endoplasmic reticulum (ER). To allow cleavage, Star transports Spi to the Golgi, where it undergoes cleavage by Rhomboid (Rho). Since some DER phenotypes are not mimicked by any of its known activating ligands, we identified an additional ligand by database searches, and termed it Keren (Krn). Krn is a functional homolog of Spi since it can rescue the spi mutant phenotype in a Rho- and Star-dependent manner. In contrast to Spi, however, Krn also possesses a Rho/Star-independent ability to undergo low-level cleavage and activate DER, as evident both in cell culture and in flies. The difference in basal activity correlates with the cellular localization of the two ligands. While Spi is retained in the ER, the retention of Krn is only partial. Examining Spi/Krn chimeric and deletion constructs implicates the Spi cytoplasmic domain in inhibiting its basal activity. Low-level activity of Krn calls for tightly regulated expression of the Krn precursor.
Figures
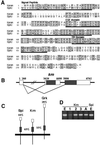
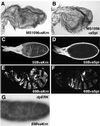
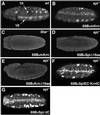
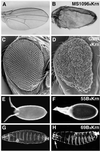
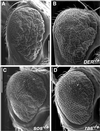
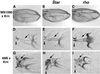
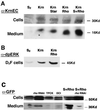
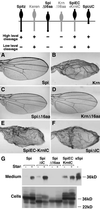
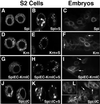
Similar articles
-
Rhomboid cleaves Star to regulate the levels of secreted Spitz.EMBO J. 2007 Mar 7;26(5):1211-20. doi: 10.1038/sj.emboj.7601581. Epub 2007 Feb 15. EMBO J. 2007. PMID: 17304216 Free PMC article.
-
Intracellular trafficking by Star regulates cleavage of the Drosophila EGF receptor ligand Spitz.Genes Dev. 2002 Jan 15;16(2):222-34. doi: 10.1101/gad.214202. Genes Dev. 2002. PMID: 11799065 Free PMC article.
-
Rhomboid and Star facilitate presentation and processing of the Drosophila TGF-alpha homolog Spitz.Genes Dev. 2000 Jan 15;14(2):177-86. Genes Dev. 2000. PMID: 10652272 Free PMC article.
-
EGF receptor signalling: the importance of presentation.Curr Biol. 2000 May 18;10(10):R388-91. doi: 10.1016/s0960-9822(00)00485-1. Curr Biol. 2000. PMID: 10837218 Review.
-
Genetic models meet trophic mechanisms: EGF family members are gliatrophins in Drosophila.Neuron. 2002 Feb 28;33(5):673-5. doi: 10.1016/s0896-6273(02)00619-0. Neuron. 2002. PMID: 11879645 Review.
Cited by
-
EGF signaling and the origin of axial polarity among the insects.Curr Biol. 2010 Jun 8;20(11):1042-7. doi: 10.1016/j.cub.2010.04.023. Epub 2010 May 13. Curr Biol. 2010. PMID: 20471269 Free PMC article.
-
EGFR/Ras/MAPK signaling mediates adult midgut epithelial homeostasis and regeneration in Drosophila.Cell Stem Cell. 2011 Jan 7;8(1):84-95. doi: 10.1016/j.stem.2010.11.026. Epub 2010 Dec 16. Cell Stem Cell. 2011. PMID: 21167805 Free PMC article.
-
Trafficking of the EGFR ligand Spitz regulates its signaling activity in polarized tissues.J Cell Sci. 2013 Oct 1;126(Pt 19):4469-78. doi: 10.1242/jcs.131169. Epub 2013 Jul 31. J Cell Sci. 2013. PMID: 23902690 Free PMC article.
-
Rhomboid cleaves Star to regulate the levels of secreted Spitz.EMBO J. 2007 Mar 7;26(5):1211-20. doi: 10.1038/sj.emboj.7601581. Epub 2007 Feb 15. EMBO J. 2007. PMID: 17304216 Free PMC article.
-
Activation of EGFR signaling by Tc-Vein and Tc-Spitz regulates the metamorphic transition in the red flour beetle Tribolium castaneum.Sci Rep. 2021 Sep 22;11(1):18807. doi: 10.1038/s41598-021-98334-9. Sci Rep. 2021. PMID: 34552169 Free PMC article.
References
-
- Baonza A., Casci,T. and Freeman,M. (2001) A primary role for the epidermal growth factor receptor in ommatidial spacing in the Drosophila eye. Curr. Biol., 11, 396–404. - PubMed
-
- Bier E., Jan,L.Y. and Jan,Y.N. (1990) Rhomboid, a gene required for dorsoventral axis establishment and peripheral nervous system development in Drosophila melanogaster. Genes Dev., 4, 190–203. - PubMed
-
- Freeman M. (2000) Feedback control of intercellular signalling in development. Nature, 408, 313–319. - PubMed
-
- Gabay L., Scholz,H., Golembo,M., Klaes,A., Shilo,B.Z. and Klambt,C. (1996) EGF receptor signaling induces pointed P1 transcription and inactivates Yan protein in the Drosophila embryonic ventral ectoderm. Development, 122, 3355–3362. - PubMed
Publication types
MeSH terms
Substances
LinkOut - more resources
Full Text Sources
Other Literature Sources
Molecular Biology Databases
Research Materials