Stress-dependent nucleolin mobilization mediated by p53-nucleolin complex formation
- PMID: 12138209
- PMCID: PMC133981
- DOI: 10.1128/MCB.22.16.6014-6022.2002
Stress-dependent nucleolin mobilization mediated by p53-nucleolin complex formation
Abstract
We recently discovered that heat shock causes nucleolin to relocalize from the nucleolus to the nucleoplasm, whereupon it binds replication protein A and inhibits DNA replication initiation. We report that nucleolin mobilization also occurs following exposure to ionizing radiation (IR) and treatment with camptothecin. Mobilization was selective in that another nucleolar marker, upstream binding factor, did not relocalize in response to IR. Nucleolin relocalization was dependent on p53 and stress, the latter initially stimulating nucleolin-p53 complex formation. Nucleolin relocalization and complex formation in vivo were independent of p53 transactivation but required the p53 C-terminal regulatory domain. Nucleolin and p53 also interact directly in vitro, with a similar requirement for p53 domains. These data indicate a novel p53-dependent mechanism in which cell stress mobilizes nucleolin for transient replication inhibition and DNA repair.
Figures
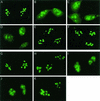
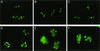
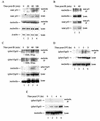
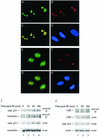
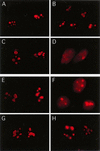
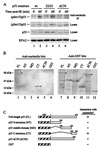
Similar articles
-
Novel checkpoint response to genotoxic stress mediated by nucleolin-replication protein a complex formation.Mol Cell Biol. 2005 Mar;25(6):2463-74. doi: 10.1128/MCB.25.6.2463-2474.2005. Mol Cell Biol. 2005. PMID: 15743838 Free PMC article.
-
Formation of a complex between nucleolin and replication protein A after cell stress prevents initiation of DNA replication.J Cell Biol. 2000 May 15;149(4):799-810. doi: 10.1083/jcb.149.4.799. J Cell Biol. 2000. PMID: 10811822 Free PMC article.
-
Specific domains of nucleolin interact with Hdm2 and antagonize Hdm2-mediated p53 ubiquitination.FEBS J. 2012 Feb;279(3):370-83. doi: 10.1111/j.1742-4658.2011.08430.x. Epub 2011 Dec 19. FEBS J. 2012. PMID: 22103682 Free PMC article.
-
Nucleolin and nucleophosmin: nucleolar proteins with multiple functions in DNA repair.Biochem Cell Biol. 2016 Oct;94(5):419-432. doi: 10.1139/bcb-2016-0068. Epub 2016 Jun 29. Biochem Cell Biol. 2016. PMID: 27673355 Review.
-
Functions of the histone chaperone nucleolin in diseases.Subcell Biochem. 2007;41:125-44. doi: 10.1007/1-4020-5466-1_7. Subcell Biochem. 2007. PMID: 17484127 Review.
Cited by
-
The lncRNA PVT1 Contributes to the Cervical Cancer Phenotype and Associates with Poor Patient Prognosis.PLoS One. 2016 May 27;11(5):e0156274. doi: 10.1371/journal.pone.0156274. eCollection 2016. PLoS One. 2016. PMID: 27232880 Free PMC article.
-
Impairment of Nucleolin Activity and Phosphorylation by a Trachylobane Diterpene from Psiadia punctulata in Cancer Cells.Int J Mol Sci. 2022 Sep 27;23(19):11390. doi: 10.3390/ijms231911390. Int J Mol Sci. 2022. PMID: 36232690 Free PMC article.
-
Pathogenesis of the erythroid failure in Diamond Blackfan anaemia.Br J Haematol. 2010 Feb;148(4):611-22. doi: 10.1111/j.1365-2141.2009.07993.x. Epub 2009 Dec 1. Br J Haematol. 2010. PMID: 19958353 Free PMC article.
-
The Nucleolus Takes Control of Protein Trafficking Under Cellular Stress.Mol Cell Pharmacol. 2010;2(5):203-212. Mol Cell Pharmacol. 2010. PMID: 21499571 Free PMC article.
-
The nucleolus exhibits an osmotically regulated gatekeeping activity that controls the spatial dynamics and functions of nucleolin.J Biol Chem. 2008 Apr 25;283(17):11823-31. doi: 10.1074/jbc.M800308200. Epub 2008 Feb 25. J Biol Chem. 2008. PMID: 18299322 Free PMC article.
References
-
- Blander, G., J. Kipnis, J. F. Leal, C. E. Yu, G. D. Schellenberg, and M. Oren. 1999. Physical and functional interaction between p53 and the Werner's syndrome protein. J. Biol. Chem. 274:29463-29469. - PubMed
-
- Budde, A., and I. Grummt. 1999. p53 represses ribosomal gene transcription. Oncogene 18:1119-1124. - PubMed
-
- Bukovsky, A., M. R. Caudle, J. A. Keenan, J. Wimalasena, J. S. Foster, N. B. Upadhyaya, and S. E. van Meter. 1995. Expression of cell cycle regulatory proteins (p53, pRb) in the human female genital tract. J. Assist. Reprod. Genet. 12:123-131. - PubMed
-
- Carrier, F., M. L. Smith, I. Bae, K. E. Kilpatrick, T. J. Lansing, C. Y. Chen, M. Engelstein, S. H. Friend, W. D. Henner, and T. M. Gilmer. 1994. Characterization of human Gadd45, a p53-regulated protein. J. Biol. Chem. 269:32672-32677. - PubMed
Publication types
MeSH terms
Substances
Grants and funding
LinkOut - more resources
Full Text Sources
Other Literature Sources
Molecular Biology Databases
Research Materials
Miscellaneous