The preprotein conducting channel at the inner envelope membrane of plastids
- PMID: 12032074
- PMCID: PMC126020
- DOI: 10.1093/emboj/21.11.2616
The preprotein conducting channel at the inner envelope membrane of plastids
Abstract
The preprotein translocation at the inner envelope membrane of chloroplasts so far involves five proteins: Tic110, Tic55, Tic40, Tic22 and Tic20. The molecular function of these proteins has not yet been established. Here, we demonstrate that Tic110 constitutes a central part of the preprotein translocation pore. Dependent on the presence of intact Tic110, radiolabelled preprotein specifically interacts with isolated inner envelope vesicles as well as with purified, recombinant Tic110 reconstituted into liposomes. Circular dichroism analysis reveals that Tic110 consists mainly of beta-sheets, a structure typically found in pore proteins. In planar lipid bilayers, recombinant Tic110 forms a cation-selective high-conductance channel with a calculated inner pore opening of 1.7 nm. Purified transit peptide causes strong flickering and a voltage-dependent block of the channel. Moreover, at the inner envelope membrane, a peptide-sensitive channel is described that shows properties basically identical to the channel formed by recombinant Tic110. We conclude that Tic110 has a distinct preprotein binding site and functions as a preprotein translocation pore at the inner envelope membrane.
Figures
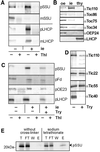
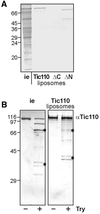
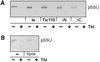
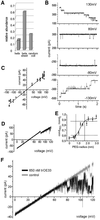
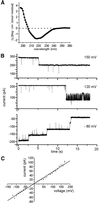
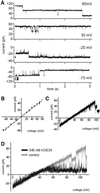
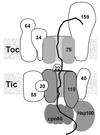
Similar articles
-
Tic20 forms a channel independent of Tic110 in chloroplasts.BMC Plant Biol. 2011 Sep 30;11:133. doi: 10.1186/1471-2229-11-133. BMC Plant Biol. 2011. PMID: 21961525 Free PMC article.
-
Tic20 and Tic22 are new components of the protein import apparatus at the chloroplast inner envelope membrane.J Cell Biol. 1998 Nov 16;143(4):991-1002. doi: 10.1083/jcb.143.4.991. J Cell Biol. 1998. PMID: 9817756 Free PMC article.
-
Tic12, a 12-kDa essential component of the translocon at the inner envelope membrane of chloroplasts in Arabidopsis.Plant Cell. 2022 Oct 27;34(11):4569-4582. doi: 10.1093/plcell/koac240. Plant Cell. 2022. PMID: 35929083 Free PMC article.
-
The TIC complex uncovered: The alternative view on the molecular mechanism of protein translocation across the inner envelope membrane of chloroplasts.Biochim Biophys Acta. 2015 Sep;1847(9):957-67. doi: 10.1016/j.bbabio.2015.02.011. Epub 2015 Feb 16. Biochim Biophys Acta. 2015. PMID: 25689609 Review.
-
Protein import into chloroplasts: the Tic complex and its regulation.Biochim Biophys Acta. 2010 Jun;1803(6):740-7. doi: 10.1016/j.bbamcr.2010.01.015. Epub 2010 Jan 25. Biochim Biophys Acta. 2010. PMID: 20100520 Review.
Cited by
-
Engineering protocells: prospects for self-assembly and nanoscale production-lines.Life (Basel). 2015 Mar 25;5(2):1019-53. doi: 10.3390/life5021019. Life (Basel). 2015. PMID: 25815781 Free PMC article. Review.
-
Nanopore unitary permeability measured by electrochemical and optical single transporter recording.Biophys J. 2005 Jun;88(6):4000-7. doi: 10.1529/biophysj.104.058255. Epub 2005 Mar 4. Biophys J. 2005. PMID: 15749773 Free PMC article.
-
Characterization of the translocon of the outer envelope of chloroplasts.J Cell Biol. 2003 Feb 17;160(4):541-51. doi: 10.1083/jcb.200210060. J Cell Biol. 2003. PMID: 12591914 Free PMC article.
-
Coexpressed subunits of dual genetic origin define a conserved supercomplex mediating essential protein import into chloroplasts.Proc Natl Acad Sci U S A. 2020 Dec 22;117(51):32739-32749. doi: 10.1073/pnas.2014294117. Epub 2020 Dec 3. Proc Natl Acad Sci U S A. 2020. PMID: 33273113 Free PMC article.
-
Protein translocation through anthrax toxin channels formed in planar lipid bilayers.Biophys J. 2004 Dec;87(6):3842-9. doi: 10.1529/biophysj.104.050864. Epub 2004 Sep 17. Biophys J. 2004. PMID: 15377524 Free PMC article.
References
-
- Bainbridge G., Gokce,I. and Lakey,J.H. (1998) Voltage gating is a fundamental feature of porin and toxin β-barrel membrane channels. FEBS Lett., 431, 305–308. - PubMed
-
- Dalmas B., Hunter,G.J. and Bannister,W.H. (1994) Prediction of protein secondary structure from circular dichroism spectra using artificial neural network techniques. Biochem. Mol. Biol. Int., 34, 17–26. - PubMed
Publication types
MeSH terms
Substances
LinkOut - more resources
Full Text Sources
Other Literature Sources