Mucus clearance as a primary innate defense mechanism for mammalian airways
- PMID: 11877463
- PMCID: PMC150901
- DOI: 10.1172/JCI15217
Mucus clearance as a primary innate defense mechanism for mammalian airways
Figures
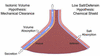
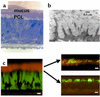
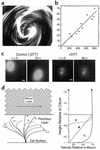
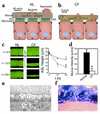
Similar articles
-
Innate immune functions of the airway epithelium.Contrib Microbiol. 2008;15:147-163. doi: 10.1159/000136349. Contrib Microbiol. 2008. PMID: 18511860 Review.
-
Mucociliary clearance--a critical upper airway host defense mechanism and methods of assessment.Curr Opin Allergy Clin Immunol. 2007 Feb;7(1):5-10. doi: 10.1097/ACI.0b013e3280114eef. Curr Opin Allergy Clin Immunol. 2007. PMID: 17218804 Review.
-
Effective mucus clearance is essential for respiratory health.Am J Respir Cell Mol Biol. 2006 Jul;35(1):20-8. doi: 10.1165/rcmb.2006-0082SF. Epub 2006 Mar 9. Am J Respir Cell Mol Biol. 2006. PMID: 16528010 Free PMC article. Review. No abstract available.
-
Model of mucociliary clearance in cystic fibrosis lungs.J Theor Biol. 2015 May 7;372:81-8. doi: 10.1016/j.jtbi.2015.02.023. Epub 2015 Mar 5. J Theor Biol. 2015. PMID: 25746843
-
Airway mucus function and dysfunction.N Engl J Med. 2010 Dec 2;363(23):2233-47. doi: 10.1056/NEJMra0910061. N Engl J Med. 2010. PMID: 21121836 Free PMC article. Review. No abstract available.
Cited by
-
Detrimental role of the airway mucin Muc5ac during ventilator-induced lung injury.Mucosal Immunol. 2013 Jul;6(4):762-75. doi: 10.1038/mi.2012.114. Epub 2012 Nov 28. Mucosal Immunol. 2013. PMID: 23187315 Free PMC article.
-
Computational Modeling Insights into Extreme Heterogeneity in COVID-19 Nasal Swab Data.Viruses. 2023 Dec 30;16(1):69. doi: 10.3390/v16010069. Viruses. 2023. PMID: 38257769 Free PMC article.
-
Acquired cilia dysfunction in chronic rhinosinusitis.Am J Rhinol Allergy. 2012 Jan-Feb;26(1):1-6. doi: 10.2500/ajra.2012.26.3716. Am J Rhinol Allergy. 2012. PMID: 22391065 Free PMC article. Review.
-
Epithelial tethering of MUC5AC-rich mucus impairs mucociliary transport in asthma.J Clin Invest. 2016 Jun 1;126(6):2367-71. doi: 10.1172/JCI84910. Epub 2016 May 16. J Clin Invest. 2016. PMID: 27183390 Free PMC article.
-
Glucocorticoid receptor and histone deacetylase-2 mediate dexamethasone-induced repression of MUC5AC gene expression.Am J Respir Cell Mol Biol. 2012 Nov;47(5):637-44. doi: 10.1165/rcmb.2012-0009OC. Epub 2012 Jul 12. Am J Respir Cell Mol Biol. 2012. PMID: 22798432 Free PMC article.
References
-
- Kilburn KH. A hypothesis for pulmonary clearance and its implications. Am Rev Respir Dis. 1968;98:449–463. - PubMed
-
- Guggino WB. Cystic fibrosis and the salt controversy. Cell. 1999;96:607–610. - PubMed
-
- Wanner A, Salathe M, O’Riordan TG. Mucociliary clearance in the airways. Am J Respir Crit Care Med. 1996;154:1868–1902. - PubMed
-
- Boucher RC. Human airway ion transport. Part 1. Am J Respir Crit Care Med. 1994;150:271–281. - PubMed
Publication types
MeSH terms
Grants and funding
LinkOut - more resources
Full Text Sources
Other Literature Sources