Removal of a single alpha-tubulin gene intron suppresses cell cycle arrest phenotypes of splicing factor mutations in Saccharomyces cerevisiae
- PMID: 11784857
- PMCID: PMC133559
- DOI: 10.1128/MCB.22.3.801-815.2002
Removal of a single alpha-tubulin gene intron suppresses cell cycle arrest phenotypes of splicing factor mutations in Saccharomyces cerevisiae
Abstract
Genetic and biochemical studies of Schizosaccharomyces pombe and Saccharomyces cerevisiae have identified gene products that play essential functions in both pre-mRNA splicing and cell cycle control. Among these are the conserved, Myb-related CDC5 (also known as Cef1p in S. cerevisiae) proteins. The mechanism by which loss of CDC5/Cef1p function causes both splicing and cell cycle defects has been unclear. Here we provide evidence that cell cycle arrest in a new temperature-sensitive CEF1 mutant, cef1-13, is an indirect consequence of defects in pre-mRNA splicing. Although cef1-13 cells harbor global defects in pre-mRNA splicing discovered through intron microarray analysis, inefficient splicing of the alpha-tubulin-encoding TUB1 mRNA was considered as a potential cause of the cef1-13 cell cycle arrest because cef1-13 cells arrest uniformly at G(2)/M with many hallmarks of a defective microtubule cytoskeleton. Consistent with this possibility, cef1-13 cells possess reduced levels of total TUB1 mRNA and alpha-tubulin protein. Removing the intron from TUB1 in cef1-13 cells boosts TUB1 mRNA and alpha-tubulin expression to near wild-type levels and restores microtubule stability in the cef1-13 mutant. As a result, cef1-13 tub1Deltai cells progress through mitosis and their cell cycle arrest phenotype is alleviated. Removing the TUB1 intron from two other splicing mutants that arrest at G(2)/M, prp17Delta and prp22-1 strains, permits nuclear division, but suppression of the cell cycle block is less efficient. Our data raise the possibility that although cell cycle arrest phenotypes in prp mutants can be explained by defects in pre-mRNA splicing, the transcript(s) whose inefficient splicing contributes to cell cycle arrest is likely to be prp mutant dependent.
Figures
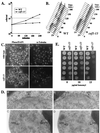
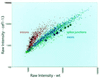
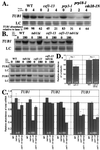
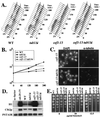
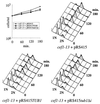
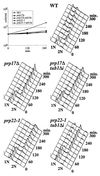
Similar articles
-
Dependence of pre-mRNA introns on PRP17, a non-essential splicing factor: implications for efficient progression through cell cycle transitions.Nucleic Acids Res. 2003 May 1;31(9):2333-43. doi: 10.1093/nar/gkg333. Nucleic Acids Res. 2003. PMID: 12711678 Free PMC article.
-
Genome-wide analysis of pre-mRNA splicing: intron features govern the requirement for the second-step factor, Prp17 in Saccharomyces cerevisiae and Schizosaccharomyces pombe.J Biol Chem. 2004 Dec 10;279(50):52437-46. doi: 10.1074/jbc.M408815200. Epub 2004 Sep 27. J Biol Chem. 2004. PMID: 15452114
-
Mutations in genes of Saccharomyces cerevisiae encoding pre-mRNA splicing factors cause cell cycle arrest through activation of the spindle checkpoint.Nucleic Acids Res. 2002 Oct 15;30(20):4361-70. doi: 10.1093/nar/gkf563. Nucleic Acids Res. 2002. PMID: 12384582 Free PMC article.
-
Pre-mRNA splicing in yeast.Trends Genet. 1991 Mar;7(3):79-85. doi: 10.1016/0168-9525(91)90276-V. Trends Genet. 1991. PMID: 2031287 Review.
-
Thirty Years with ERH: An mRNA Splicing and Mitosis Factor Only or Rather a Novel Genome Integrity Protector?Cells. 2023 Oct 13;12(20):2449. doi: 10.3390/cells12202449. Cells. 2023. PMID: 37887293 Free PMC article. Review.
Cited by
-
Dependence of pre-mRNA introns on PRP17, a non-essential splicing factor: implications for efficient progression through cell cycle transitions.Nucleic Acids Res. 2003 May 1;31(9):2333-43. doi: 10.1093/nar/gkg333. Nucleic Acids Res. 2003. PMID: 12711678 Free PMC article.
-
The Saccharomyces cerevisiae gene CDC40/PRP17 controls cell cycle progression through splicing of the ANC1 gene.Nucleic Acids Res. 2004 May 7;32(8):2529-40. doi: 10.1093/nar/gkh574. Print 2004. Nucleic Acids Res. 2004. PMID: 15133121 Free PMC article.
-
SON controls cell-cycle progression by coordinated regulation of RNA splicing.Mol Cell. 2011 Apr 22;42(2):185-98. doi: 10.1016/j.molcel.2011.03.014. Mol Cell. 2011. PMID: 21504830 Free PMC article.
-
Structural requirement of Ntc77 for spliceosome activation and first catalytic step.Nucleic Acids Res. 2014 Oct 29;42(19):12261-71. doi: 10.1093/nar/gku914. Epub 2014 Oct 7. Nucleic Acids Res. 2014. PMID: 25294830 Free PMC article.
-
Loss of splicing factor ASF/SF2 induces G2 cell cycle arrest and apoptosis, but inhibits internucleosomal DNA fragmentation.Genes Dev. 2005 Nov 15;19(22):2705-14. doi: 10.1101/gad.1359305. Epub 2005 Oct 31. Genes Dev. 2005. PMID: 16260492 Free PMC article.
References
-
- Albright, S. R., and R. Tjian. 2000. TAFs revisited: more data reveal new twists and confirm old ideas. Gene 242:1–13. - PubMed
-
- Apone, L. M., C. M. Virbasius, J. C. Reese, and M. R. Green. 1996. Yeast TAF(II)90 is required for cell-cycle progression through G2/M but not for general transcription activation. Genes Dev. 10:2368–2380. - PubMed
-
- Archer, J. E., L. R. Vega, and F. Solomon. 1995. Rbl2p, a yeast protein that binds to beta-tubulin and participates in microtubule function in vivo. Cell 82:425–434. - PubMed
Publication types
MeSH terms
Substances
Grants and funding
LinkOut - more resources
Full Text Sources
Other Literature Sources
Molecular Biology Databases
Research Materials