Mitochondrial respiratory electron carriers are involved in oxidative stress during heat stress in Saccharomyces cerevisiae
- PMID: 11713283
- PMCID: PMC100011
- DOI: 10.1128/MCB.21.24.8483-8489.2001
Mitochondrial respiratory electron carriers are involved in oxidative stress during heat stress in Saccharomyces cerevisiae
Abstract
In the present study we sought to determine the source of heat-induced oxidative stress. We investigated the involvement of mitochondrial respiratory electron transport in post-diauxic-phase cells under conditions of lethal heat shock. Petite cells were thermosensitive, had increased nuclear mutation frequencies, and experienced elevated levels of oxidation of an intracellular probe following exposure to a temperature of 50 degrees C. Cells with a deletion in COQ7 leading to a deficiency in coenzyme Q had a much more severe thermosensitivity phenotype for these oxidative endpoints following heat stress compared to that of petite cells. In contrast, deletion of the external NADH dehydrogenases NDE1 and NDE2, which feed electrons from NADH into the electron transport chain, abrogated the levels of heat-induced intracellular fluorescence and nuclear mutation frequency. Mitochondria isolated from COQ7-deficient cells secreted more than 30 times as much H(2)O(2) at 42 as at 30 degrees C, while mitochondria isolated from cells simultaneously deficient in NDE1 and NDE2 secreted no H(2)O(2). We conclude that heat stress causes nuclear mutations via oxidative stress originating from the respiratory electron transport chains of mitochondria.
Figures
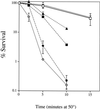
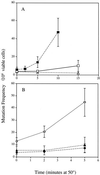
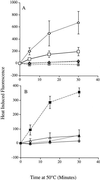

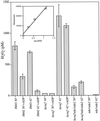
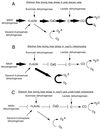
Similar articles
-
nde1 deletion improves mitochondrial DNA maintenance in Saccharomyces cerevisiae coenzyme Q mutants.Biochem J. 2013 Feb 1;449(3):595-603. doi: 10.1042/BJ20121432. Biochem J. 2013. PMID: 23116202
-
Demethoxy-Q, an intermediate of coenzyme Q biosynthesis, fails to support respiration in Saccharomyces cerevisiae and lacks antioxidant activity.J Biol Chem. 2004 Jun 18;279(25):25995-6004. doi: 10.1074/jbc.M400001200. Epub 2004 Apr 12. J Biol Chem. 2004. PMID: 15078893
-
Cytotoxic and genotoxic consequences of heat stress are dependent on the presence of oxygen in Saccharomyces cerevisiae.J Bacteriol. 2001 Aug;183(15):4580-7. doi: 10.1128/JB.183.15.4580-4587.2001. J Bacteriol. 2001. PMID: 11443093 Free PMC article.
-
Pro-oxidant mitochondrial matrix-targeted ubiquinone MitoQ10 acts as anti-oxidant at retarded electron transport or proton pumping within Complex I.Int J Biochem Cell Biol. 2009 Aug-Sep;41(8-9):1697-707. doi: 10.1016/j.biocel.2009.02.015. Epub 2009 Mar 3. Int J Biochem Cell Biol. 2009. PMID: 19433311
-
The biochemical, pathophysiological, and medical aspects of ubiquinone function.Ann N Y Acad Sci. 1998 Nov 20;854:394-409. doi: 10.1111/j.1749-6632.1998.tb09919.x. Ann N Y Acad Sci. 1998. PMID: 9928447 Review.
Cited by
-
Effects of increased temperature on metabolic activity and oxidative stress in the first life stages of marble trout (Salmo marmoratus).Fish Physiol Biochem. 2015 Aug;41(4):1005-14. doi: 10.1007/s10695-015-0065-6. Epub 2015 May 3. Fish Physiol Biochem. 2015. PMID: 25935664
-
Respiratory metabolism and calorie restriction relieve persistent endoplasmic reticulum stress induced by calcium shortage in yeast.Sci Rep. 2016 Jun 16;6:27942. doi: 10.1038/srep27942. Sci Rep. 2016. PMID: 27305947 Free PMC article.
-
Exogenous calcium improves viability of biocontrol yeasts under heat stress by reducing ROS accumulation and oxidative damage of cellular protein.Curr Microbiol. 2012 Aug;65(2):122-7. doi: 10.1007/s00284-012-0133-4. Epub 2012 May 6. Curr Microbiol. 2012. PMID: 22562600
-
Sugar metabolism, redox balance and oxidative stress response in the respiratory yeast Kluyveromyces lactis.Microb Cell Fact. 2009 Aug 30;8:46. doi: 10.1186/1475-2859-8-46. Microb Cell Fact. 2009. PMID: 19715615 Free PMC article.
-
Isotope-reinforced polyunsaturated fatty acids protect yeast cells from oxidative stress.Free Radic Biol Med. 2011 Jan 1;50(1):130-8. doi: 10.1016/j.freeradbiomed.2010.10.690. Epub 2010 Oct 16. Free Radic Biol Med. 2011. PMID: 20955788 Free PMC article.
References
-
- Ames B N, Shigenaga M K, Hagen T M. Mitochondrial decay in aging. Biochim Biophys Acta. 1995;1271:165–170. - PubMed
-
- Anderson R F. Energetics of the one-electron reduction steps of riboflavin, FMN and FAD to their fully reduced forms. Biochim Biophys Acta. 1983;722:158–162. - PubMed
-
- Bakker B M, Overkamp K M, van Maris A J, Kotter P, Luttik M A, van Dijken J P, Pronk J T. Stoichiometry and compartmentation of NADH metabolism in Saccharomyces cerevisiae. FEMS Microbiol Rev. 2001;25:15–37. - PubMed
Publication types
MeSH terms
Substances
Grants and funding
LinkOut - more resources
Full Text Sources
Molecular Biology Databases