The target of rapamycin signaling pathway regulates mRNA turnover in the yeast Saccharomyces cerevisiae
- PMID: 11694578
- PMCID: PMC60265
- DOI: 10.1091/mbc.12.11.3428
The target of rapamycin signaling pathway regulates mRNA turnover in the yeast Saccharomyces cerevisiae
Abstract
The target of rapamycin (TOR) signaling pathway is an important mechanism by which cell growth is regulated by nutrient availability in eukaryotes. We provide evidence that the TOR signaling pathway controls mRNA turnover in Saccharomyces cerevisiae. During nutrient limitation (diauxic shift) or after treatment with rapamycin (a specific inhibitor of TOR), multiple mRNAs were destabilized, whereas the decay of other mRNAs was unaffected. Our findings suggest that the regulation of mRNA decay by the TOR pathway may play a significant role in controlling gene expression in response to nutrient depletion. The inhibition of the TOR pathway accelerated the major mRNA decay mechanism in yeast, the deadenylation-dependent decapping pathway. Of the destabilized mRNAs, two different responses to rapamycin were observed. Some mRNAs were destabilized rapidly, while others were affected only after prolonged exposure. Our data suggest that the mRNAs that respond rapidly are destabilized because they have short poly(A) tails prematurely either as a result of rapid deadenylation or reduced polyadenylation. In contrast, the mRNAs that respond slowly are destabilized by rapid decapping. In summary, the control of mRNA turnover by the TOR pathway is complex in that it specifically regulates the decay of some mRNAs and not others and that it appears to control decay by multiple mechanisms.
Figures
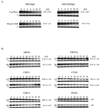
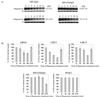
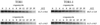
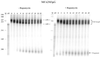
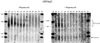
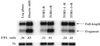
Similar articles
-
Regulation of ribosome biogenesis by the rapamycin-sensitive TOR-signaling pathway in Saccharomyces cerevisiae.Mol Biol Cell. 1999 Apr;10(4):987-1000. doi: 10.1091/mbc.10.4.987. Mol Biol Cell. 1999. PMID: 10198052 Free PMC article.
-
Two TOR complexes, only one of which is rapamycin sensitive, have distinct roles in cell growth control.Mol Cell. 2002 Sep;10(3):457-68. doi: 10.1016/s1097-2765(02)00636-6. Mol Cell. 2002. PMID: 12408816
-
Elucidating TOR signaling and rapamycin action: lessons from Saccharomyces cerevisiae.Microbiol Mol Biol Rev. 2002 Dec;66(4):579-91, table of contents. doi: 10.1128/MMBR.66.4.579-591.2002. Microbiol Mol Biol Rev. 2002. PMID: 12456783 Free PMC article. Review.
-
TOR kinase domains are required for two distinct functions, only one of which is inhibited by rapamycin.Cell. 1995 Jul 14;82(1):121-30. doi: 10.1016/0092-8674(95)90058-6. Cell. 1995. PMID: 7606777
-
Nutrient signaling through TOR kinases controls gene expression and cellular differentiation in fungi.Curr Top Microbiol Immunol. 2004;279:53-72. doi: 10.1007/978-3-642-18930-2_4. Curr Top Microbiol Immunol. 2004. PMID: 14560951 Review.
Cited by
-
Profiling condition-specific, genome-wide regulation of mRNA stability in yeast.Proc Natl Acad Sci U S A. 2005 Dec 6;102(49):17675-80. doi: 10.1073/pnas.0503803102. Epub 2005 Nov 29. Proc Natl Acad Sci U S A. 2005. PMID: 16317069 Free PMC article.
-
2',3'-cAMP, 3'-AMP, and 2'-AMP inhibit human aortic and coronary vascular smooth muscle cell proliferation via A2B receptors.Am J Physiol Heart Circ Physiol. 2011 Aug;301(2):H391-401. doi: 10.1152/ajpheart.00336.2011. Epub 2011 May 27. Am J Physiol Heart Circ Physiol. 2011. PMID: 21622827 Free PMC article.
-
Target of rapamycin (TOR) in nutrient signaling and growth control.Genetics. 2011 Dec;189(4):1177-201. doi: 10.1534/genetics.111.133363. Genetics. 2011. PMID: 22174183 Free PMC article. Review.
-
Repression of mitochondrial translation, respiration and a metabolic cycle-regulated gene, SLF1, by the yeast Pumilio-family protein Puf3p.PLoS One. 2011;6(5):e20441. doi: 10.1371/journal.pone.0020441. Epub 2011 May 31. PLoS One. 2011. PMID: 21655263 Free PMC article.
-
Reduction in ribosomal protein synthesis is sufficient to explain major effects on ribosome production after short-term TOR inactivation in Saccharomyces cerevisiae.Mol Cell Biol. 2011 Feb;31(4):803-17. doi: 10.1128/MCB.01227-10. Epub 2010 Dec 13. Mol Cell Biol. 2011. PMID: 21149576 Free PMC article.
References
Publication types
MeSH terms
Substances
Grants and funding
LinkOut - more resources
Full Text Sources
Molecular Biology Databases