The nucleoporin Nup60p functions as a Gsp1p-GTP-sensitive tether for Nup2p at the nuclear pore complex
- PMID: 11535617
- PMCID: PMC2196189
- DOI: 10.1083/jcb.200101007
The nucleoporin Nup60p functions as a Gsp1p-GTP-sensitive tether for Nup2p at the nuclear pore complex
Abstract
The nucleoporins Nup60p, Nup2p, and Nup1p form part of the nuclear basket structure of the Saccharomyces cerevisiae nuclear pore complex (NPC). Here, we show that these necleoporins can be isolated from yeast extracts by affinity chromatography on karyopherin Kap95p-coated beads. To characterize Nup60p further, Nup60p-coated beads were used to capture its interacting proteins from extracts. We find that Nup60p binds to Nup2p and serves as a docking site for Kap95p-Kap60p heterodimers and Kap123p. Nup60p also binds Gsp1p-GTP and its guanine nucleotide exchange factor Prp20p, and functions as a Gsp1p guanine nucleotide dissociation inhibitor by reducing the activity of Prp20p. Yeast lacking Nup60p exhibit minor defects in nuclear export of Kap60p, nuclear import of Kap95p-Kap60p-dependent cargoes, and diffusion of small proteins across the NPC. Yeast lacking Nup60p also fail to anchor Nup2p at the NPC, resulting in the mislocalization of Nup2p to the nucleoplasm and cytoplasm. Purified Nup60p and Nup2p bind each other directly, but the stability of the complex is compromised when Kap60p binds Nup2p. Gsp1p-GTP enhances by 10-fold the affinity between Nup60p and Nup2p, and restores binding of Nup2p-Kap60p complexes to Nup60p. The results suggest a dynamic interaction, controlled by the nucleoplasmic concentration of Gsp1p-GTP, between Nup60p and Nup2p at the NPC.
Figures
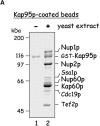
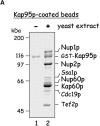
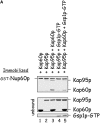
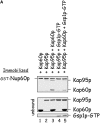
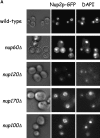
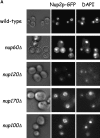
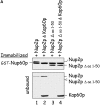
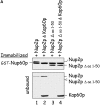
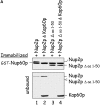
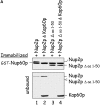
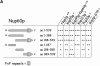
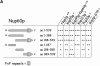
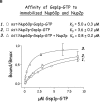
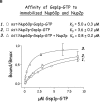
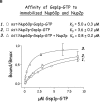
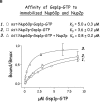
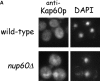
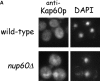
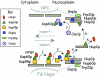
Similar articles
-
Accelerating the rate of disassembly of karyopherin.cargo complexes.J Biol Chem. 2002 May 17;277(20):18161-72. doi: 10.1074/jbc.M112306200. Epub 2002 Feb 26. J Biol Chem. 2002. PMID: 11867631
-
Nup2p, a yeast nucleoporin, functions in bidirectional transport of importin alpha.Mol Cell Biol. 2000 Nov;20(22):8468-79. doi: 10.1128/MCB.20.22.8468-8479.2000. Mol Cell Biol. 2000. PMID: 11046143 Free PMC article.
-
Nup2p dynamically associates with the distal regions of the yeast nuclear pore complex.J Cell Biol. 2001 Jun 25;153(7):1465-78. doi: 10.1083/jcb.153.7.1465. J Cell Biol. 2001. PMID: 11425876 Free PMC article.
-
Nucleocytoplasmic transport in yeast: a few roles for many actors.Biochem Soc Trans. 2010 Feb;38(Pt 1):273-7. doi: 10.1042/BST0380273. Biochem Soc Trans. 2010. PMID: 20074073 Review.
-
Review: transport of tRNA out of the nucleus-direct channeling to the ribosome?J Struct Biol. 2000 Apr;129(2-3):288-94. doi: 10.1006/jsbi.2000.4226. J Struct Biol. 2000. PMID: 10806079 Review.
Cited by
-
Nuclear pore basket proteins are tethered to the nuclear envelope and can regulate membrane curvature.Dev Cell. 2015 May 4;33(3):285-98. doi: 10.1016/j.devcel.2015.02.017. Dev Cell. 2015. PMID: 25942622 Free PMC article.
-
Meiotic cellular rejuvenation is coupled to nuclear remodeling in budding yeast.Elife. 2019 Aug 9;8:e47156. doi: 10.7554/eLife.47156. Elife. 2019. PMID: 31397671 Free PMC article.
-
Structural basis for Nup2p function in cargo release and karyopherin recycling in nuclear import.EMBO J. 2003 Oct 15;22(20):5358-69. doi: 10.1093/emboj/cdg538. EMBO J. 2003. PMID: 14532109 Free PMC article.
-
New twist to nuclear import: When two travel together.Commun Integr Biol. 2013 Jul 1;6(4):e24792. doi: 10.4161/cib.24792. Epub 2013 May 3. Commun Integr Biol. 2013. PMID: 23940825 Free PMC article.
-
Kap121p-mediated nuclear import is required for mating and cellular differentiation in yeast.Mol Cell Biol. 2002 Apr;22(8):2544-55. doi: 10.1128/MCB.22.8.2544-2555.2002. Mol Cell Biol. 2002. PMID: 11909949 Free PMC article.
References
-
- Allen, N., L. Huang, A. Burlingame, and M. Rexach. 2001. Proteomic analysis of nucleoporin interacting proteins. J. Biol. Chem. 276:29268–29274. - PubMed
-
- Baker, D., L. Hicke, M. Rexach, M. Schleyer, and R. Schekman. 1988. Reconstitution of SEC gene product-dependent intercompartmental protein transport. Cell. 54:335–344. - PubMed
-
- Booth, J.W., K.D. Belanger, M.I. Sannella, and L.I. Davis. 1999. The yeast nucleoporin Nup2p is involved in nuclear export of importin α/Srp1p. J. Biol. Chem. 274:32360–32367. - PubMed
-
- Brachmann, C.B., A. Davies, G.J. Cost, E. Caputo, J. Li, P. Hieter, and J.D. Boeke. 1998. Designer deletion strains derived from Saccharomyces cerevisiae S288C: a useful set of strains and plasmids for PCR-mediated gene disruption and other applications. Yeast. 14:115–132. - PubMed
Publication types
MeSH terms
Substances
LinkOut - more resources
Full Text Sources
Molecular Biology Databases