Comparisons of CapG and gelsolin-null macrophages: demonstration of a unique role for CapG in receptor-mediated ruffling, phagocytosis, and vesicle rocketing
- PMID: 11514591
- PMCID: PMC2196452
- DOI: 10.1083/jcb.200101113
Comparisons of CapG and gelsolin-null macrophages: demonstration of a unique role for CapG in receptor-mediated ruffling, phagocytosis, and vesicle rocketing
Abstract
Capping the barbed ends of actin filaments is a critical step for regulating actin-based motility in nonmuscle cells. The in vivo function of CapG, a calcium-sensitive barbed end capping protein and member of the gelsolin/villin family, has been assessed using a null Capg allele engineered into mice. Both CapG-null mice and CapG/gelsolin double-null mice appear normal and have no gross functional abnormalities. However, the loss of CapG in bone marrow macrophages profoundly inhibits macrophage colony stimulating factor-stimulated ruffling; reintroduction of CapG protein by microinjection fully restores this function. CapG-null macrophages also demonstrate approximately 50% impairment of immunoglobulin G, and complement-opsonized phagocytosis and lanthanum-induced vesicle rocketing. These motile functions are not impaired in gelsolin-null macrophages and no additive effects are observed in CapG/gelsolin double-null macrophages, establishing that CapG function is distinct from, and does not overlap with, gelsolin in macrophages. Our observations indicate that CapG is required for receptor-mediated ruffling, and that it is a major functional component of macrophage phagocytosis. These primary effects on macrophage motile function suggest that CapG may be a useful target for the regulation of macrophage-mediated inflammatory responses.
Figures
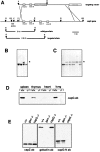
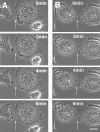
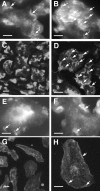
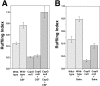
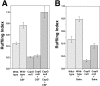
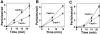
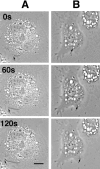
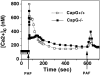
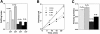
Similar articles
-
Gelsolin, a protein that caps the barbed ends and severs actin filaments, enhances the actin-based motility of Listeria monocytogenes in host cells.Infect Immun. 1998 Aug;66(8):3775-82. doi: 10.1128/IAI.66.8.3775-3782.1998. Infect Immun. 1998. PMID: 9673261 Free PMC article.
-
Clinical significance of gelsolin-like actin-capping protein expression in oral carcinogenesis: an immunohistochemical study of premalignant and malignant lesions of the oral cavity.BMC Cancer. 2008 Feb 1;8:39. doi: 10.1186/1471-2407-8-39. BMC Cancer. 2008. PMID: 18237446 Free PMC article.
-
CapG(-/-) mice have specific host defense defects that render them more susceptible than CapG(+/+) mice to Listeria monocytogenes infection but not to Salmonella enterica serovar Typhimurium infection.Infect Immun. 2003 Nov;71(11):6582-90. doi: 10.1128/IAI.71.11.6582-6590.2003. Infect Immun. 2003. PMID: 14573680 Free PMC article.
-
Gelsolin superfamily proteins: key regulators of cellular functions.Cell Mol Life Sci. 2004 Oct;61(19-20):2614-23. doi: 10.1007/s00018-004-4225-6. Cell Mol Life Sci. 2004. PMID: 15526166 Review.
-
Gelsolin: the tail of a molecular gymnast.Cytoskeleton (Hoboken). 2013 Jul;70(7):360-84. doi: 10.1002/cm.21117. Epub 2013 Jun 27. Cytoskeleton (Hoboken). 2013. PMID: 23749648 Review.
Cited by
-
Prognostic significance of MCM2, Ki-67 and gelsolin in non-small cell lung cancer.BMC Cancer. 2006 Aug 1;6:203. doi: 10.1186/1471-2407-6-203. BMC Cancer. 2006. PMID: 16882345 Free PMC article.
-
Dynamics of the CapG actin-binding protein in the cell nucleus studied by FRAP and FCS.Chromosome Res. 2008;16(3):427-37. doi: 10.1007/s10577-008-1234-6. Chromosome Res. 2008. PMID: 18461482
-
Yersinia effector YopO uses actin as bait to phosphorylate proteins that regulate actin polymerization.Nat Struct Mol Biol. 2015 Mar;22(3):248-55. doi: 10.1038/nsmb.2964. Epub 2015 Feb 9. Nat Struct Mol Biol. 2015. PMID: 25664724 Free PMC article.
-
CapG promoted nasopharyngeal carcinoma cell motility involving Rho motility pathway independent of ROCK.World J Surg Oncol. 2022 Oct 19;20(1):347. doi: 10.1186/s12957-022-02808-7. World J Surg Oncol. 2022. PMID: 36258216 Free PMC article.
-
A CapG gain-of-function mutant reveals critical structural and functional determinants for actin filament severing.EMBO J. 2006 Oct 4;25(19):4458-67. doi: 10.1038/sj.emboj.7601323. Epub 2006 Sep 14. EMBO J. 2006. PMID: 16977317 Free PMC article.
References
-
- Amatruda, J.F., J.F. Cannon, K. Tatchell, C. Hug, and J.A. Cooper. 1990. Disruption of the actin cytoskeleton in yeast capping protein mutants. Nature. 344:352–354. - PubMed
-
- Arnaudeau, S., W.L. Kelley, J.V. Walsh, Jr., and N. Demaurex. 2001. Mitochondria recycle calcium to the endoplasmic reticulum and prevent the depletion of neighboring ER regions. J. Biol. Chem. 276:29430–29439. - PubMed
Publication types
MeSH terms
Substances
Grants and funding
LinkOut - more resources
Full Text Sources
Molecular Biology Databases
Research Materials
Miscellaneous