A novel extracellular calcium sensing mechanism in voltage-gated potassium ion channels
- PMID: 11404399
- PMCID: PMC6762739
- DOI: 10.1523/JNEUROSCI.21-12-04143.2001
A novel extracellular calcium sensing mechanism in voltage-gated potassium ion channels
Abstract
Potassium (K(+)) channels influence neurotransmitter release, burst firing rate activity, pacing, and critical dampening of neuronal circuits. Internal and external factors that further modify K(+) channel function permit fine-tuning of neuronal circuits. Human ether-à-go-go-related gene (HERG) K(+) channels are unusually sensitive to external calcium concentration ([Ca(2+)](o)). Small changes in [Ca(2+)](o) shift the voltage dependence of channel activation to more positive membrane potentials, an effect that cannot be explained by nonspecific surface charge screening or channel pore block. The HERG-calcium concentration-response relationship spans the physiological range for [Ca(2+)](o). The modulatory actions of calcium are attributable to differences in the Ca(2+) affinity between rested and activated channels. Adjacent extracellular, negatively charged amino acids (E518 and E519) near the S4 voltage sensor influence both channel gating and Ca(2+) dependence. Neutralization of these charges had distinct effects on channel gating and calcium sensitivity. A change in the degree of energetic coupling between these amino acids on transition from closed to activated channel states reveals movement in this region during channel gating and defines a molecular mechanism for protein state-dependent ligand interactions. The results suggest a novel extracellular [Ca(2+)](o) sensing mechanism coupled to allosteric changes in channel gating and a mechanism for fine-tuning cell repolarization.
Figures
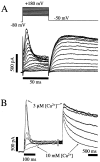
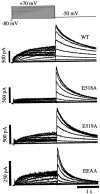
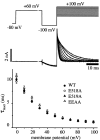
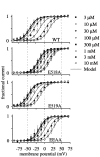
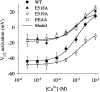
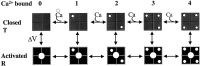
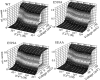
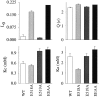
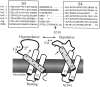
Similar articles
-
Human ether-à-go-go-related gene K+ channel gating probed with extracellular ca2+. Evidence for two distinct voltage sensors.J Gen Physiol. 1999 Apr;113(4):565-80. doi: 10.1085/jgp.113.4.565. J Gen Physiol. 1999. PMID: 10102937 Free PMC article.
-
HERG K(+) channel activity is regulated by changes in phosphatidyl inositol 4,5-bisphosphate.Circ Res. 2001 Dec 7;89(12):1168-76. doi: 10.1161/hh2401.101375. Circ Res. 2001. PMID: 11739282
-
Dynamic control of deactivation gating by a soluble amino-terminal domain in HERG K(+) channels.J Gen Physiol. 2000 Jun;115(6):749-58. doi: 10.1085/jgp.115.6.749. J Gen Physiol. 2000. PMID: 10828248 Free PMC article.
-
Physiology of EAG K+ channels.J Membr Biol. 2001 Jul 1;182(1):1-15. doi: 10.1007/s00232-001-0031-3. J Membr Biol. 2001. PMID: 11426295 Review. No abstract available.
-
Voltage sensor movement in the hERG K+ channel.Novartis Found Symp. 2005;266:46-52; discussion 52-6, 95-9. doi: 10.1002/047002142x.ch5. Novartis Found Symp. 2005. PMID: 16050261 Review.
Cited by
-
Differences between ion binding to eag and HERG voltage sensors contribute to differential regulation of activation and deactivation gating.Channels (Austin). 2007 Nov-Dec;1(6):429-37. doi: 10.4161/chan.1.6.5760. Epub 2008 Feb 19. Channels (Austin). 2007. PMID: 18690045 Free PMC article.
-
Modification of hERG1 channel gating by Cd2+.J Gen Physiol. 2010 Aug;136(2):203-24. doi: 10.1085/jgp.201010450. J Gen Physiol. 2010. PMID: 20660661 Free PMC article.
-
Ether-à-gogo-related gene (erg1) potassium channels shape the dark response of horizontal cells in the mammalian retina.Pflugers Arch. 2009 Jun;458(2):359-77. doi: 10.1007/s00424-008-0609-z. Epub 2008 Nov 8. Pflugers Arch. 2009. PMID: 18998156
-
Negative charges in the transmembrane domains of the HERG K channel are involved in the activation- and deactivation-gating processes.J Gen Physiol. 2003 Jun;121(6):599-614. doi: 10.1085/jgp.200308788. J Gen Physiol. 2003. PMID: 12771194 Free PMC article.
-
Extracellular sodium interacts with the HERG channel at an outer pore site.J Gen Physiol. 2002 Oct;120(4):517-37. doi: 10.1085/jgp.20028589. J Gen Physiol. 2002. PMID: 12356854 Free PMC article.
References
-
- Arcangeli A, Rosati B, Cherubini A, Crociani O, Fontana L, Ziller C, Wanke E, Olivotto M. HERG- and IRK-like inward rectifier currents are sequentially expressed during neuronal development of neural crest cells and their derivatives. Eur J Neurosci. 1997;9:2596–2604. - PubMed
-
- Bezanilla F. The voltage sensor in voltage-dependent ion channels. Physiol Rev. 2000;80:555–592. - PubMed
-
- Bianchi L, Wible B, Arcangeli A, Taglialatela M, Morra F, Castaldo P, Crociani O, Rosati B, Faravelli L, Olivotto M, Wanke E. Herg encodes a K+ current highly conserved in tumors of different histogenesis: a selective advantage for cancer cells. Cancer Res. 1998;58:815–822. - PubMed
Publication types
MeSH terms
Substances
Grants and funding
LinkOut - more resources
Full Text Sources
Miscellaneous